Introduction
Materials and Methods
Reagents
Preparation of AR
Cell culture
MTT assay
Cytokine assay
Western blot analysis
Luciferase reporter gene assay
Caspase-1 activity assay
Statistical analysis
Results
Effect of AR on cell viability in PMACI- stimulated HMC-1 cells
Effect of AR on inflammatory cytokine secretion in PMACI- stimulated HMC-1 cells
Effect of AR on NF-κB activation in PMACI-stimulated HMC-1 cells
Effect of AR on caspase-1 activation in PMACI -stimulated HMC-1 cells
Discussion
Introduction
Allergic inflammatory diseases including asthma, atopic dermatitis and allergic rhinitis, have become global health problems (Hawiger, 2001). Allergic inflammation plays an important role in allergic diseases. Mast cells are crucial effector cells and are associated with allergic disorders (Modena et al., 2016). Specifically, activated mast cells release preformed and synthesized inflammatory cytokines including interleukin (IL)-6, IL-8 and tumor necrosis factor (TNF)-a which contribute to the development of allergic inflammation (Furue and Kadono, 2017). It has been shown that mast cells are present in larger numbers in AD lesional skin and mast cell depletion could interfere with allergic rhinitis (Lim et al., 2018). Thus, these findings suggest that the prevention of mast cell activation and cytokine production could be a potent treatment strategy for allergic inflammation-related diseases.
Nuclear factor-kappa B (NF-kB) is a transcription factor that regulates numerous genes involved in immune and allergic inflammation (Lee et al., 2020). An increase in NF-kB activity associated with the secretion of high levels of inflammatory cytokines was shown to be involved in allergic inflammatory responses (Gilmore and Garbati, 2011). Emerging evidence has shown that NF-kB represents a potential target for treating allergic diseases. It was reported that the inhibition of NF-kB activation diminished the influx of inflammatory cells and reduced allergic inflammation (Birrell et al., 2005). Caspase-1 is a member of the family of caspases and is associated with apoptosis and inflammation. The activation of caspase-1 induced an allergic inflammatory response by increasing inflammatory cytokines and the recruitment of inflammatory cells (Kim et al., 2016). Caspase-1 deficiency was reported to reduce inflammation in an allergic rhinitis mice model (Martin et al., 2013). These results suggest caspase-1 activation as an attractive target for the treatment of inflammatory diseases.
Traditional medicines are commonly used as a complementary and alternative therapy for various allergic diseases (Song et al., 2016). Although herbal medicines have long been effectively employed, their precise mechanism is not completely understood. Adenophorae Radix (AR) has been widely used as natural food as well as traditional medicine. AR has been used for the prevention of cough, sputum, asthma and cancer (Kim and Kang, 2017; Kim et al., 2008; Li et al., 2016). Despite previous studies demonstrating the pharmacological activity of AR, information on the precise mechanism of AR on mast cell-mediated allergic inflammation remains limited. To find a possible explanation for the anti-allergic inflammation mechanism of AR, we investigated the effect of AR on the expression of inflammatory cytokines as well as the activation of NF-kB/caspase-1 in phorbol 12-myristate 13-acetate plus calcium ionophore A23187 (PMACI)-stimulated human mast cells (HMC-1).
Materials and Methods
Reagents
We purchased phorbol 12-myristate 13-acetate (PMA), calcium Ionophore A23187, avidin peroxidase (AP), dimethyl sulfoxide (DMSO), 3-(4,5-dimethylthiazol-2-yl)-2,5-diphenyltetrazolium bromide (MTT) and phosphate buffered saline (PBS) from the Sigma Chemical Co. (St. Louis, MO, USA). Iscove’s Modified Dulbecco’s Medium (IMDM) was procured from Gibco BRL (Grand Island, NY, USA). Fetal bovine serum (FBS), the bicinchoninic acid (BCA) assay kit (Cat:23227) and enhanced chemiluminescence (ECL) kit (Cat:32106) were obtained from Thermo Fisher Scientific Inc. (Somerset, NJ, USA). Anti-human TNF-α (Cat:555212)/IL-6 (Cat:550799)/ IL-1β(Cat:557966) /IL-8(Cat:550999) antibodies (Abs) assay were procured from BD Biosciences (San Diego, CA, USA). NF-κB (sc-515045), IκB-α (sc-71291) and histone Abs were purchased from Santa Cruz Biotechnology, Inc. (Santa Cruz, CA, USA).
Preparation of AR
We purchased the dried root of AR from the Human herb (Gyeongbuk, Korea). A voucher specimen (no. WD2019-10) was authenticated by Dr. Jong-Hyun Lee, the Botanical Garden of Dongduk Women’s University and deposited at Wonkwang Digital University. The AR dried root (200 g) was pulverized into a fine powder and extracted in 2 L of a 70% aqueous ethanol solution at room temperature for 24 h, then concentrated under vacuum. The ethanol extract was filtered (Whatman, GD/X, USA), lyophilized (Sunileyela, EYELA-FDU-1200, Japan), and kept at 4℃ (yield, 13.7%). The samples were dissolved in PBS and filtered through a 0.22 ㎛ syringe filter.
Cell culture
Human mast cell line, HMC-1, cells were grown in IMDM medium supplemented with 100 IU/mL penicillin, 100 ㎍/mL streptomycin, and 10% heat-inactivated FBS at 37℃ in a humidified incubator under 5% CO2. The HMC-1 cells were stimulated with PMA (50 nM) plus A23187 (1 ㎍/mL).
MTT assay
To test the cell viability at each concentration of AR, the MTT colorimetric assay was performed. Briefly, the cells were incubated with AR (0.01, 0.1 and 1 ㎎/mL) for 12 h and 50 µL of MTT (5 ㎎/mL) solution was subsequently added and incubated for 4 h. Next, the crystallized formazan was dissolved in 1 mL of DMSO and the absorbance of the wells was read at 540 ㎚ (Molecular Devices, Sunnyvale, CA, USA).
Cytokine assay
The production of TNF-α, IL-6, IL-1β and IL-8 was evaluated by modified enzyme-linked immunosorbent assays (ELISA) as previously described (Kim et al., 2010). Briefly, 96-well plates were coated with anti-human TNF-α, IL-6, IL-1β and IL-8 monoclonal Abs and incubated overnight at 4℃. After additional washes, the samples or TNF-α, IL-6, IL-1β and IL-8 standard solution was added and incubated for 2 h. The plates were exposed to biotinylated Abs and incubated for 2 h. After washing the plates, AP and ABTS substrates containing H2O2 were sequentially added. Color development was evaluated at 405 ㎚ by a microplate reader.
Western blot analysis
Nuclear extracts were prepared using NE-PER Nuclear and Cytoplasmic Extraction Reagents. After protein quantification using the BCA protein assay kit, the supernatant was mixed with sample buffer, separated by gel electrophoresis, and transferred to membranes. The membranes were then blocked with 5% skimmed milk and subsequently reacted with the primary Abs. After washing, the membranes were incubated with secondary Abs for 1 h. After washing with 0.1% PBST, the protein bands were visualized using an ECL detection system.
Luciferase reporter gene assay
NF-κB transcriptional activity was measured using NF-κB promoter luciferase assay, as previously described (Sakamoto et al., 2018). The cells were transfected with NF-κB-luc DNA and refreshed with complete IMDM media. The transfected cells were seeded in 6-well plates and treated with AR before PMACI stimulation for another 2 h. Luciferase activity was determined using the Dual-Glo Luciferase Assay System following the manufacturer’s instructions (Promega, Madison, WI, USA).
Caspase-1 activity assay
Caspase-1 activity was assayed using a caspase colorimetric assay kit (R&D System Inc., Minneapolis, MN, USA, Cat: K111-100) according to the manufacturer’s protocols. After protein quantification using the BCA protein assay kit, the protein supernatant was incubated with 50 µL of reaction buffer and 5 µL of caspase substrate at 37℃ for 2 h. The absorbance was measured using a plate reader at a wavelength of 405 ㎚.
Statistical analysis
The results are shown as the mean ± S.D and each experiment was performed at least three times. The statistical analyses were performed using an independent t-test and ANOVA with a Tukey post hoc test. P < 0.05 was considered significant.
Results
Effect of AR on cell viability in PMACI- stimulated HMC-1 cells
To investigate the anti-allergic inflammatory effect of AR, the human mast cell line, HMC-1, was employed in this study. First, The cells were pretreated with or without AR (0.01, 0.1 and 1 ㎎/mL) for 1 h prior to stimulation with PMACI for 8 h and the cytotoxic effects of AR was evaluated using an MTT assay. The result showed that no cell cytotoxicity of AR was observed (Fig. 1A).
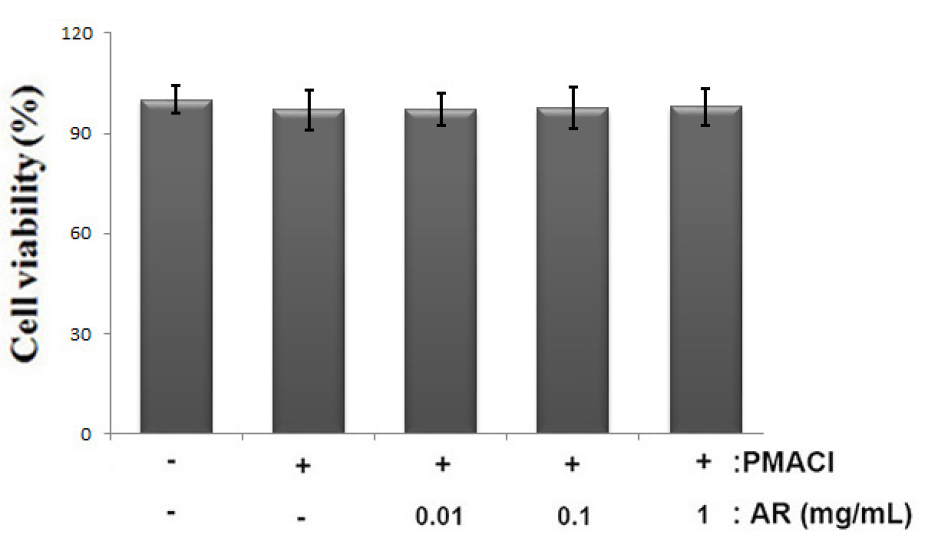
Fig. 1
Effect of AR on cell viability in PMACI-stimulated HMC-1 cells. (A) Cells (1 × 105cells/well) were treated with AR (0.01, 0.1 and 1 ㎎/mL) for 12 h and cell viability was analyzed by the MTT assay. The values are presented as the mean ± SD of independent experiments (#P < 0.05 vs. control, *P < 0.05 vs. PMACI alone).
Effect of AR on inflammatory cytokine secretion in PMACI- stimulated HMC-1 cells
The suppression of inflammatory cytokine levels is one of the widely accepted therapeutic strategies for allergic inflammation (Furue and Kadono, 2017). Therefore, we evaluated the regulatory effect of AR on TNF-α, IL-6, IL-1β and IL-8 production in PMACI-stimulated HMC-1 cells. The cells were pretreated with or without AR (0.01, 0.1 and 1 ㎎/mL) for 1 h prior to stimulation with PMACI for 8 h. The results showed that PMACI alone markedly induced the secretion of TNF-α, IL-6, IL-1β and IL-8 by PMACI-stimulated HMC-1 cells. However, AR suppressed the PMACI-induced TNF-α, IL-6, IL-1β and IL-8 production in a dose-dependent manner (Fig. 2). The maximal inhibition of TNF-α, IL-6, IL-1β and IL-8 secretion by AR (1 ㎎/mL) was approximately 35.2% (p < 0.05), 31.7% (p < 0.05), 30.4% (p < 0.05), and 34.7% (p < 0.05), respectively.
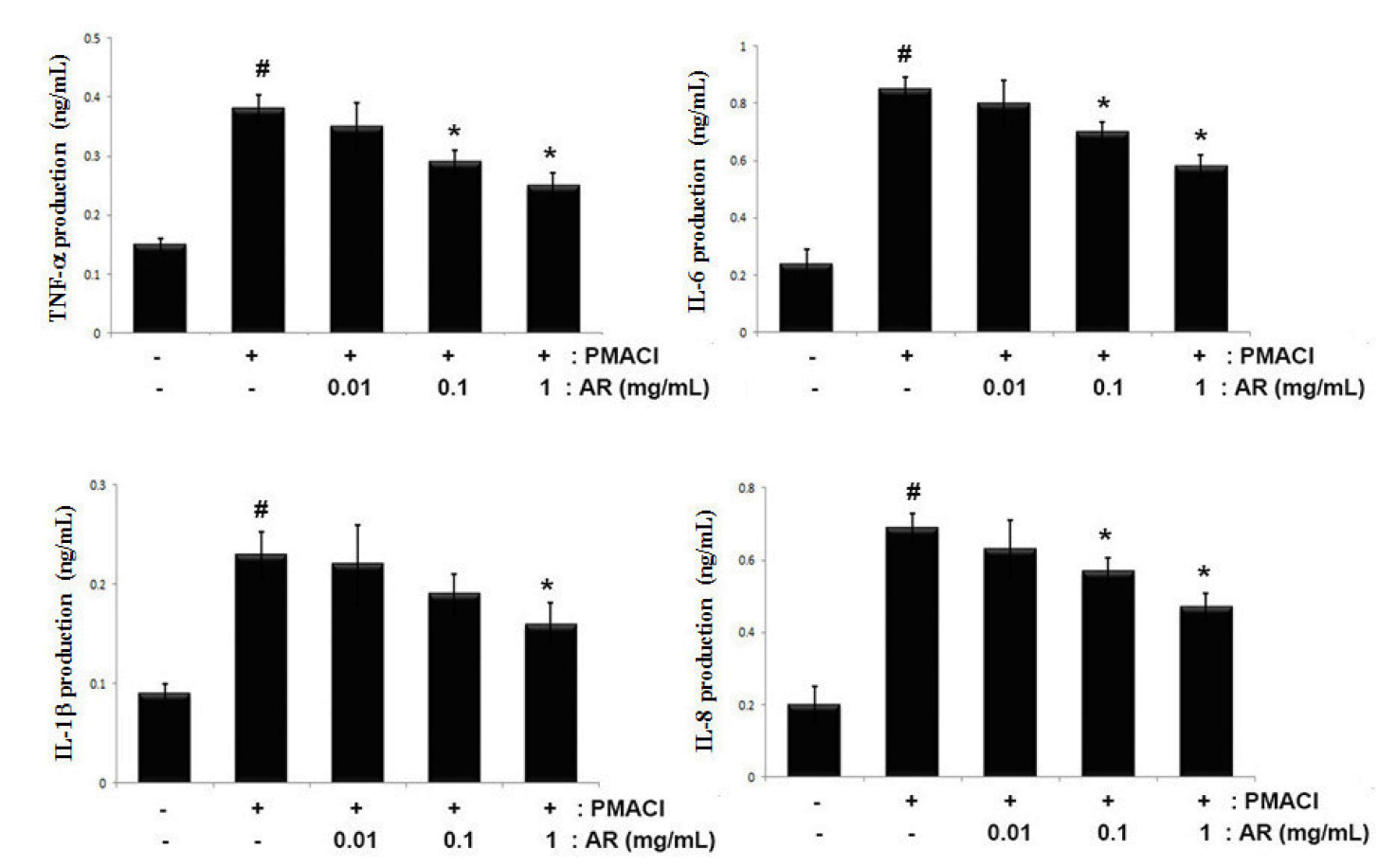
Fig. 2
Effects of AR on TNF-α, IL-6, IL-1β and IL-8 production in PMACI-stimulated HMC-1 cells. Cells (5 × 105cells/well) were pretreated with or without AR (0.01, 0.1 and 1 ㎎/mL) for 1 h prior to stimulation with PMACI (50 mM PMA + 1 ㎎/mL A23187) for 8 h. The levels of TNF-α, IL-6, IL-1β and IL-8 in the indicated groups were measured by ELISA. The values are presented as the mean ± SD of independent experiments (#P < 0.05 vs. control, *P < 0.05 vs. PMACI alone).
Effect of AR on NF-κB activation in PMACI-stimulated HMC-1 cells
As the suppression of NF-kB activation has been linked to anti-allergic inflammation, we theorized that the molecular mechanism of AR might be mediated via the suppression of NF-kB activation. Generally, because NF-kB activation requires the IκB-α degradation in cytosol and nuclear translocation of the RelA/p65 subunit of NF-kB, we evaluated the effects of AR on the IκB-α degradation and NF-κB (RelA/ p65) translocation in nuclear by Western blot analysis. As shown in Fig. 3A, PMACI induced the IκB-α degradation compared with the untreated control. However, AR (0.1 and 1 ㎎/mL) suppressed the PMACI-induced IκB-α degradation in cytosol. The levels of Rel/p65 in the nuclear fraction were increased in PMACI-stimulated cells, but AR reduced the enhanced nuclear levels of Rel/p65. The relative levels of IκB-α (in the cytosol) and NF-kB (in the nucleus) are shown in Fig. 3B. Moreover, to evaluate the effect of AR on the promoter activity of NF-kB, a luciferase reporter assay was conducted. As illustrated in Fig. 3C, AR attenuated the NF-kB-driven luciferase activity in PMACI-stimulated HMC-1 cells.
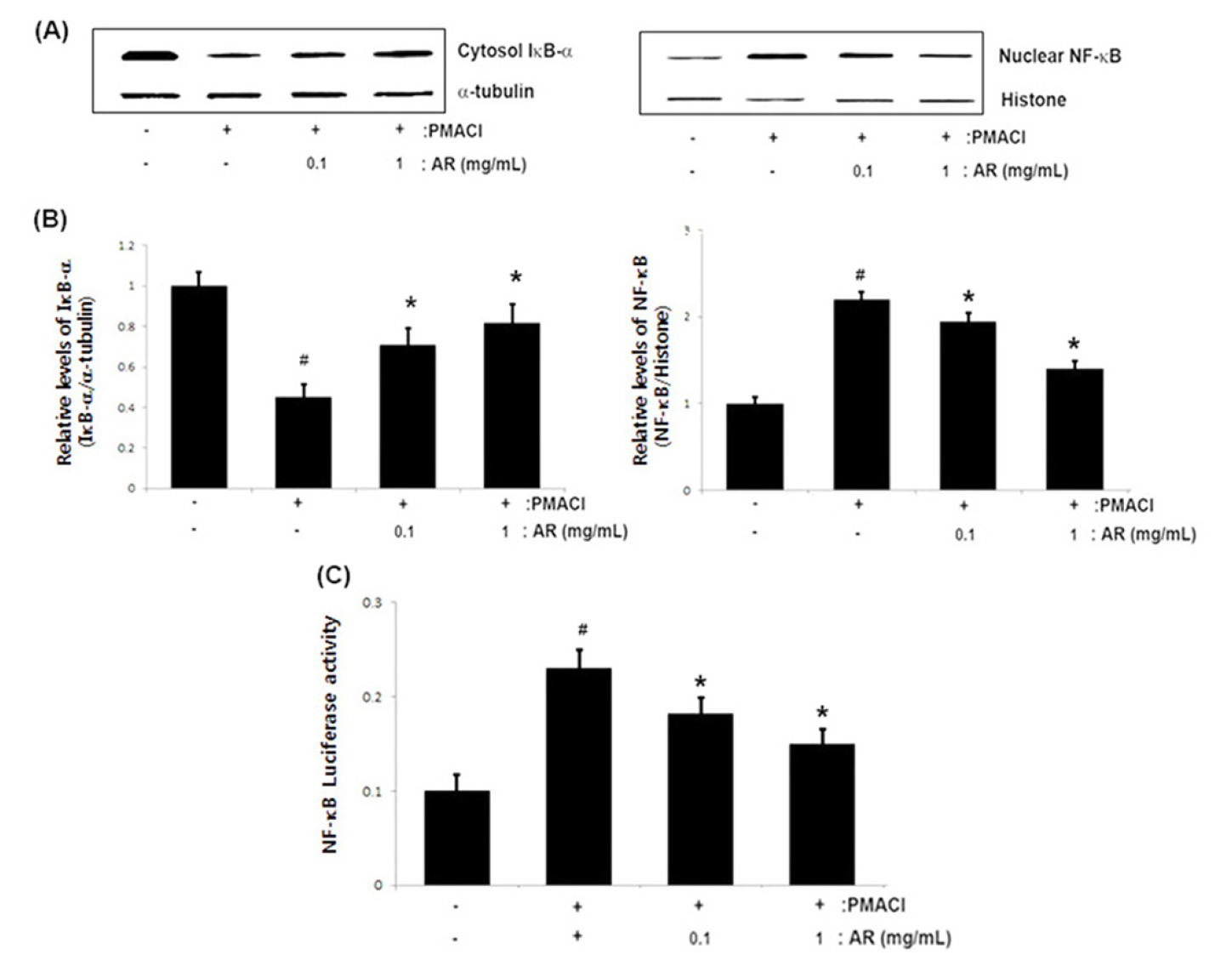
Fig. 3
Effect of AR on the NF-κB activation in PMACI-stimulated HMC-1 cells. Cells (6 × 106cells/well) were pre-treated with AR (0.1 and 1 ㎎/mL) for 1 h and then stimulated with PMACI (50 mM PMA + 1 ㎎/mL A23187) for 2 h. (A) Cytosolic and nuclear extracts were prepared and evaluated for IκB-α in cytosol and NF-κB (RelA/p65) in nucelar by Western blot analysis. (B) The relative levels of IκB-α and NF-κB (RelA/p65) are presented. (C) Luciferase activity was determined using a luciferase assay system kit following the manufacturer’s instructions. The values are presented as the mean ± SD of independent experiments (#P < 0.05 vs. control, *P < 0.05 vs. PMACI alone).
Effect of AR on caspase-1 activation in PMACI -stimulated HMC-1 cells
The activation of caspase-1 induces an inflammatory response via the stimulation of inflammatory cytokines (Han et al., 2017). To determine the molecular mechanism of AR involved in allergic inflammation, we investigated the effects of AR on PMACI-induced caspase-1 activity using a caspase-1 assay kit. The results showed that the enhanced caspase-1 activity induced by PMACI was significantly reduced by AR in a dose-dependent manner (Fig. 4). The maximal inhibition of caspase-1 activity by AR (1 ㎎/mL) was approximately 40.8% (p < 0.05).
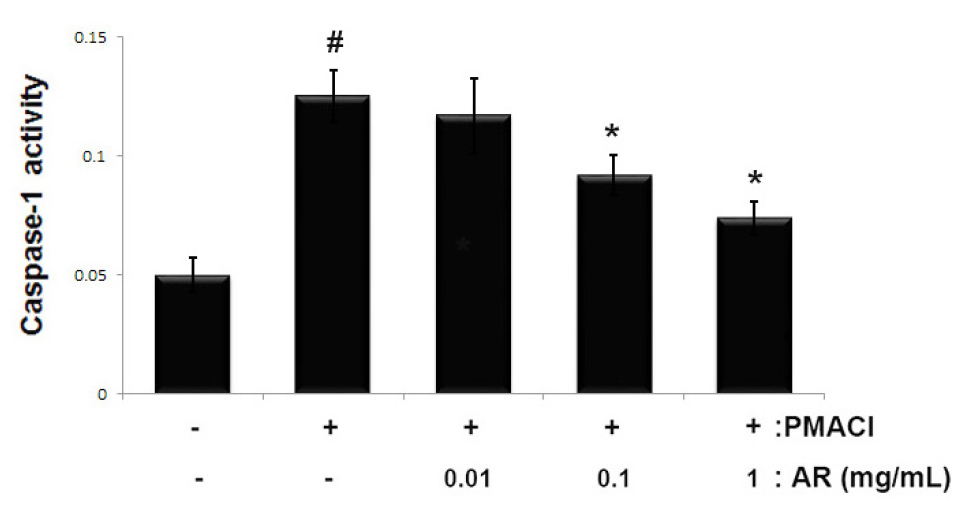
Fig. 4
Effect of AR on caspase-1 activation in PMACI-stimulated HMC-1. Cells (5 × 105 cells/well) were pretreated with or without AR (0.01, 0.1 and 1 ㎎/mL) for 1 h prior to stimulation with PMACI (50 mM PMA + 1 ㎎/mL A23187) for 4 h. The enzymatic activity of caspase-1 was measured using a caspase-1 colorimetric assay. The values are presented as the mean ± SD of independent experiments (#P < 0.05 vs. control, *P < 0.05 vs. PMACI alone).
Discussion
Traditional medicines have received revived interest as alternative treatment for variety of diseases. Although traditional medicines have long been effectively employed, their precise mechanisms are not completely understood. In this study, we demonstrated the beneficial effects and molecular mechanisms of AR in mast cell-mediated allergic inflammation in vitro model. The findings of this study showed that AR effectively attenuated the PMACI-induced production of TNF-α, IL-6, IL-1β and IL-8 in stimulated HMC-1cells. Additionally, we showed that the inhibitory mechanisms of AR on allergic inflammation arose from suppressing the activation of NF-kB/caspase-1 in PMACI- stimulated HMC-1cells.
Allergic inflammation plays a central role in allergic diseases and involves the action of multiple factors within a complex network (Edwards et al., 2017). Mast cells are crucial effector cells and are associated with allergic disorders (Caughey, 2016). specifically, activated mast cells release preformed and synthesized inflammatory cytokines which contribute to the development of allergic inflammation. Emerging evidence has shown that mast cells are present in larger numbers in patients with allergic inflammatory diseases and plays an integral role in allergic inflammation pathogenesis (Theoharides et al., 2019). Thus, these findings suggest that preventing mast cell activation and cytokine production may be a potent treatment strategy for allergic inflammation-related diseases. In this study, we demonstrated that AR attenuated the release of TNF-α, IL-6, IL-1β and IL-8 in PMACI-simulated HMC-1 cells. The inhibition of TNF-α, IL-6, IL-1β and IL-8 by AR (1 ㎎/mL) was approximately 35.2% (p < 0.05), 31.7% (p < 0.05), 30.4% (p < 0.05), and 34.7% (p < 0.05), respectively. These results collectively demonstrate that AR exerted an anti-allergic inflammatory effect by suppressing inflammatory cytokine release from mast cells.
NF-κB regulates the transcription of numerous genes involved in allergic inflammation (Lee et al., 2020). In the inactive state (under normal condition), NF-κB/ IκB complexes are sequestered in the cytoplasm. During the inflammatory process, the IκB kinase (IKK) complex phosphorylate and degrades IκB protein (DiDonato et al., 2012). As a result, free NF-κB is translocated into the nucleus where it can combine with the target genes and activate various inflammatory factors (Mitchell and Carmody, 2018). Several studies have shown that increases of inflammatory mediators were associated with increased caspase-1 activation (Han et al., 2017). The activation of caspsase-1 was shown to induce an allergic inflammatory response by causing an increase in inflammatory cytokines and the recruitment of inflammatory cells (Bouchier-Hayes and Martin, 2004). It has been reported that caspase-1 deficiency reduced inflammation in an allergic rhinitis mice model (Kuida et al., 1995). Additionally, it was revealed that activation of caspasse-1 induced NF-kB and MAPK-signaling pathways (Lamkanfi et al., 2004; Siegmund et al., 2001). Based on these results, the suppression of NF-kB/caspase-1 activation was identified as an anti-inflammatory strategy. Therefore, to identify the regulatory mechanism of AR on allergic inflammation, we assessed whether AR could suppress the activation of NF-kB/caspase-1 in PMACI-simulated HMC-1 cells. The results demonstrated that AR inhibited PMACI-induced the IκB-α degradation in cytosol and NF-kB translocation into the nucleus. Additionally, we observed that AR attenuated the PMACI-induced caspase-1 activation in a dose-dependent manner. From this, we hypothesized that AR exerted mast cell-mediated anti-inflammatory mechanisms via the inhibition of NF-κB/caspase-1 activation. A model of the anti-allergic inflammatory mechanism of AR has been provided in Fig. 5. Although AR attenuated NF-kB/caspase-1 activation, the effect of AR on another pathway including MAPK or PI3K/Akt-signaling was not elucidated. Therefore, further study is necessary to clarify the role of AR in other pathways in mast cell-mediated allergic inflammation.
In conclusion, AR was shown to regulate mast cell-mediated allergic inflammation by suppressing inflammatory cytokines levels. We demonstrated that the anti-allergic inflammatory mechanism of AR could be attributed to the inhibition of the activation of NF-kB/caspase-1 in PMCI-stimulated mast cells. Our study provides experimental evidence that AR may be a useful candidate for treating allergic inflammatory-related diseases.