Introduction
Materials and Methods
Experimental materials
DPPH radical scavenging activity
ABTS radical scavenging activity
Analysis of total phenolic compounds
Cell culture
Cell viability
Immunoblotting
Reverse transcription-Polymerase chain reaction (RT-PCR)
Quantitative PCR Analysis
Statistical analysis
Results and Discussion
Introduction
These days, research is being actively conducted to develop pharmaceuticals and cosmetics from natural materials-that have anti-tumor, antioxidant, and anti-aging properties-for the treatment and alleviation of various diseases (Yoo et al., 2005). Antioxidants are crucial for protecting against oxidative damage that can lead to conditions, such as Alzheimer’s disease, cancer, and chronic inflammation (Dinstel et al., 2013; Hussain et al., 2003; Sen et al., 2010). Antioxidants can regulate the levels of various radicals by converting them into stable forms with paired electrons, to maintain redox balance and to prevent reactive oxygen species (ROS) from causing serious damage to biomolecules (Circu and Aw, 2010). Plant-sourced natural antioxidants such as vitamin C, vitamin E, carotenes, phenolics, flavonoids, phytates, and phytoestrogens play a critical role by interfering with the oxidation process by reacting with free radicals, chelating catalytic metals and scavenging oxygen in biological systems (Pisoschi et al., 2009; Prasad et al., 2010). Therefore, there is a growing interest in searching for alternative, natural, and safer sources of antioxidants. Additionally, ROS plays an important role in tumor initiation by mediating DNA damage through oxidative stress (Han et al., 2016), and the damaged cells activate complex signaling networks, to either reverse DNA damage or promote cell death in response to DNA damage (Roos et al., 2016). p53-induced DNA damage through an activated kinase plays a pivotal role in activating the DNA repair proteins (Shibata and Jeggo, 2014). Kinase-activated γ-H2AX plays an important role in DNA damage because it aggregates other genes that repair DNA damage in response to oxidative stress in cells (Paull et al., 2000). Broussonetia kazinoki Siebold (B. kazinoki) is a deciduous shrub that belongs to the family Moraceae (Lee, 2003). B. kazinoki have been used for manufacturing Korean paper for a long time in the East, and have been used as a medicine for the treatment of burns and acne (Lee et al., 2014). Methanol extracts of B. kazinoki have been found to possess tyrosinase inhibitory activity (Baek et al., 2009) and anti-inflammatory effects (Bae et al., 2011). Especially, the anticancer effects of the methanol extract of the root bark of B. kazinoki have been studied in A549 cell lines (Kim et al., 2016). In many previous studies, valuable functions of use have been examined in derives of B. kazinoki. The purpose of this study was to investigate the protective effect of DNA damage based on antioxidant activity.
Materials and Methods
Experimental materials
Peeled root (PR), Root bark (RB), Peeled stem (PS), and Stem bark (SB) of B. kazinoki Siebold (voucher number: JWU-042) were obtained from the Goesan Korean paper Experience Museum, 233, Wonpung-ro, Yeonpung-myeon, Goesan-gun, Chungcheongbuk-do, Republic of Korea. All other chemicals were purchased from Sigma-Aldrich (St. Louis, MO, USA) unless otherwise specified.
DPPH radical scavenging activity
DPPH radical scavenging activity was measured in accordance with the Bondet method (Bondet et al., 1997), with minor modifications. Absorbance was measured using a UV/Visible spectrophotometer (Xma-3000PC, HumanCorp, Seoul, Korea) at 515 ㎚.
ABTS radical scavenging activity
ABTS radical scavenging activity was measured as described by Van den Berg et al. (1999) with some modifications. Absorbance was measured using a UV/Visible spectrophotometer at 732 ㎚.
Analysis of total phenolic compounds
Total phenolic compounds were analyzed in accordance with the Folin-Denis method (AOAC, 1995). The supernatants were measured at 725 nm using a UV/Visible spectrophotometer. Total phenolic compounds were expressed as ㎎ tannic acid equivalents (g/㎎ TAE).
Cell culture
NIH/3T3 cells were purchased from American Type Culture Collection (ATCC, Manassas, VA, USA) and cultured in DMEM media supplemented with 10% (v/v) bovine calf serum, 100 U/mL penicillin, and 100 ㎍/mL streptomycin. The cells were maintained at 37℃ under conditions of humidified atmosphere containing 5% CO2.
Cell viability
Cell viability was measured by CellTiter 96® AQueous One solution, cell proliferation assay (Promega, Madison, WI, USA) and the manufacturer's protocols were followed. After the experiment, the absorbance was measured at 490 ㎚ to determine cell viability.
Immunoblotting
The cells were lysed in radioimmunoprecipitation assay buffer (Thermo Scientific, Waltham, MA, USA) supplemented with protease inhibitor cocktail (Sigma-Aldrich). The protein concentration in the sample was determined using the Bradford protein assay (Bio-Rad, Hercules, CA, USA). The proteins were then separated on a 10% SDS-PAGE gel and transferred to a polyvinylidene fluoride membrane (Bio-Rad). The membranes were blocked with 5% bovine serum albumin (BSA) in Tris-buffered saline (TBS) containing 1% Tween 20 (TBS-T) and then incubated with specific primary antibodies in 3% BSA at 4℃ overnight. Subsequently, the blots were washed three times with TBS-T and incubated with horseradish peroxidase-conjugated immunoglobulin G for 1 h. Chemiluminescence was detected using the ECL Western blotting substrate (Bio-Rad) and visualized using Chemi-Doc (Bio-rad, Hercules, CA, USA).
Reverse transcription-Polymerase chain reaction (RT-PCR)
The total RNA was extracted from B16F10 cells using Nucleo Spin® RNA Plus (Macherey-Nagel, Düren, Germany), and cDNA was synthesized using Rever Tra Ace -α- (Toyobo, Osaka, Japan) in accordance with the manufacturer’s protocol. PCR was carried out using Quick Taq® HS Dye Mix (Toyobo).
Quantitative PCR Analysis
The cDNAs was then used as a template for quantitative polymerase chain reaction (qPCR) using Quanti Tect® SYBR Green PCR Kit (Qiagen) as per the manufacturer’s instructions. To quantify the mRNA expression, qPCR analysis was performed on a 7500 Real-Time PCR system (Applied Biosystems, CA, USA). qPCR primers were designed using the Primer 3 program (Table 1). Datasets were analyzed using ABI 7500 Software 2.3 (Applied Biosystems).
Table 1. Primer sequences
Statistical analysis
Statistical analysis was carried out using SPSS 18.0 (SPSS, Inc., Chicago, IL, USA). All experiments were performed at least three times. The data obtained from experiments carried out in triplicates are shown as the mean ± SD. Differences were considered statistically significant when the P-value was < 0.05.
Results and Discussion
Reactive oxygen species (ROS) can damage DNA either directly or indirectly (Wiseman and Halliwell, 1996; Yu et al., 2016). Indirect damage is caused by the reaction of ROS with biomolecules such as proteins and lipids, and other components in the cell. Direct damage occurs through one of the following routes: single electron transfer (SET), hydrogen atom transfer (HAT), or radical adduct formation (RAF) (Minko et al., 2009). In particular, ROS can produce not only single, but multiple lesions, defects, and mutations in DNA molecules, which may lead to the formation of cross-linked products (Bellon et al., 2002; Box et al., 1997; Crean et al., 2008; Leinisch et al., 2017; Uvaydov et al., 2014; Zeng and Wang, 2007; Zhang and Wang, 2003). Phenolic compounds present in plants have electron-donating potential due to their resonance structure, and their antioxidant potential can relatively reduce the ROS levels (Valko et al., 2005). For quantifying the antioxidant activity, DPPH and ABTS radicals scavenging activity assays were conducted. These radicals are reactive and react with nucleotides or whole DNA molecules (Reiter et al., 2010). Increased antioxidant capacity can protect against DNA damage, thereby leading to the prevention of carcinogenesis, mutagenesis, and various genetic disorders (Galano et al., 2015; Reiter et al., 2014). Scavenging activity against DPPH and ABTS radicals were confirmed by measuring the antioxidant activity of each part of B. kazinoki separately (Fig. 1). DPPH and ABTS radicals are the most widely used and stable chromogen compounds to measure the antioxidant activity of biological material (Que et al., 2006). DPPH is a free radical and ABTS is a cationic radical complex with potassium persulfate. There is a difference in the reaction between each radical and the antioxidant (Meir et al., 1995). The inhibitory concentration 50 (IC50) values of DPPH for each extract were, 33.3 ± 9.6 ㎍/mL (PR), 20.1 ± 2.3 ㎍/mL (RB), 60.0 ± 19.6 ㎍/mL (PS), and 18.8 ± 6.6 ㎍/mL (SB). The IC50 values of ABTS were 5.3 ± 0.0 ㎍/mL (PR), 4.4 ± 1.2 ㎍/mL (RB), 7.0 ± 0.4 ㎍/mL (PS), and 6.2 ± 0.6 ㎍/mL (SB). Analysis of the total phenolic compounds from the different parts from B. kazinoki (Fig. 2) revealed that the amount of total phenolic compounds in PR, RB, PS, and SB was 7.4 ± 0.2, 5.2 ± 0.1, 5.6 ± 0.1, and 8.1 ± 0.2 ㎎/g TAE, respectively.
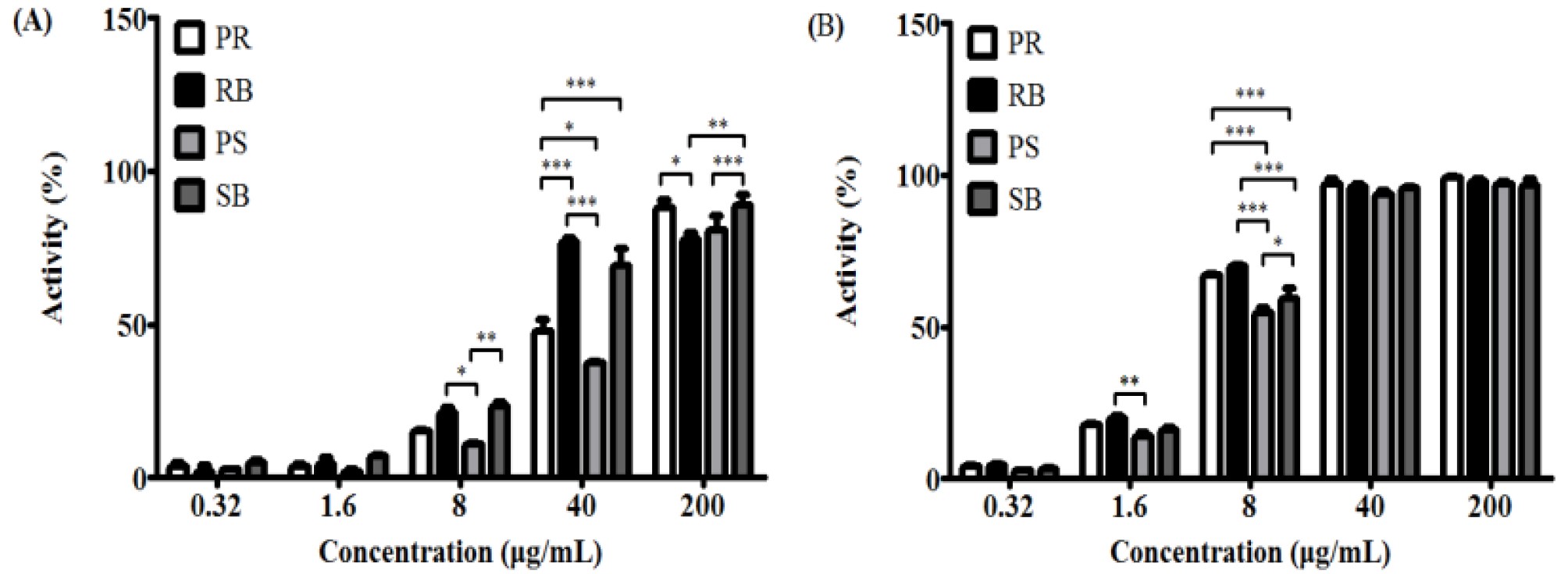
Fig. 1.
DPPH radical (A) and ABTS radical (B) scavenging activity of different parts from Broussonetia kazinoki Siebold. Values are expressed as the means ± SD of three independent experiments. PR; peeled root, RB; root bark, PS; peeled stem, and SB; stem bark. *p < 0.05, **p < 0.01, ***p < 0.001 as compared to each group.
Medicinal plants reportedly protect biomolecules, and their various bioactivities are attributed to the presence of phenolic compounds. Phenolic compounds are involved in deactivating ROS or inhibiting their formation (Alvarez-Idaboy and Galano, 2012). Phenolic compounds play an important role in inhibiting ROS-induced DNA damage (Attaguile et al., 2000). The cytotoxicity of B. kazinoki was observed at 77.7-138.2% at 25 and 50 ㎍/mL. There were no statistically significant, but high cytotoxicity was seen at 25.7-40.6% at 100 ㎍/mL and 9.0-22.3% at 200 ㎍/mL (Fig. 3). ROS plays an important role in carcinogenesis through oxidative stress-induced DNA damage (Kong et al., 2001), and is directly related to apoptosis of cells (Simon et al., 2000). H2AX, the most important factor in the DNA damage pathway (Fernandez-Capetillo et al., 2004), and p53, a tumor suppressor, are closely related to DNA damage caused by high ROS levels (Sablina et al., 2005). Oxidative stress caused by elevated ROS causes phosphorylation of ATM/ ATR kinases, cell cycle checkpoint kinases (Chk2 / Chk1), tumor suppressor p53, and phosphorylated histone γ-H2AX foci. Thus, the expression of H2AX and p53 is used as an indicator of DNA damage (Maréchal and Zou, 2013). Therefore, alleviation of ROS is very important, and antioxidants offer protection from these radicals in the body (Maxwell, 1995). To evaluate the expression of p-p53, NIH/3T3 cells were pre-treated with each extract and oxidative damage was measured. The expression of p-p53 was increased in the treated cells compared to that in the untreated group. As a result, the ROS inhibitory effects of extracts from different parts of B. kazinoki in the context of DNA damage were assessed. In oxidative stress-induced NIH/3T3 cells, it was observed that PR, RB, PS, and SB inhibited the expression of p-p53 gene, as seen by immunoblotting (Fig. 4), RT-PCR, and qPCR analyses (Fig. 5). Compared to the basal level, oxidative damage significantly increased the p-p53 expression by 239.7 ± 16.0%. However, PR, RB, PS, and SB inhibited the expression of p-p53 (195.6 ± 8.1%, 236.2 ± 11.1%, 198.0 ± 19.5%, and 107.6 ± 18.1% at 50 ㎍/mL, respectively). A similar pattern was observed when p53 mRNA levels were quantified thereby confirming the results (Fig. 5D). Further, compared to the basal level, oxidative damage significantly increased the mRNA levels of p53 by 128.3 ± 2.8%. However, PR, RB, PS, and SB inhibited the mRNA levels of p53 (85.4 ± 2.7%, 102.1 ± 4.6%, 65.8 ± 5.1%, and 64.1 ± 6.5% at 50 ㎍/mL, respectively). Moreover, qPCR analysis revealed that the mRNA levels of p53 were upregulated by 1.77 ± 0.08-fold in oxidative damage-induced NIH/3T3 cells relative to those in the untreated group. The mRNA levels of p53 were downregulated by treatment with PR, RB, PS, and SB by 1.13 ± 0.06-fold, 1.40 ± 0.08-fold, 0.65 ± 0.04-fold, and 0.54 ± 0.03-fold at 50 ㎍/mL, respectively. To evaluate γ-H2AX expression, NIH/3T3 cells were pre-treated with each extract and oxidative damage. The expression of γ-H2AX increased compared to that in the untreated group. As a result of the inhibitory effect of the extracts from different parts from B. kazinoki on DNA damage, PR, RB, PS, and SB inhibited the γ-H2AX expression in oxidative stress-induced NIH/3T3 cells, as seen by immunoblotting (Fig. 4), RT-PCR and qPCR analyses (Fig. 5). Compared to the basal level, oxidative damage significantly increased γ-H2AX expression by 144.7 ± 6.1%. However, PR, RB, PS, and SB inhibited the expression of γ-H2AX (38.1 ± 2.8%, 133.3 ± 4.2%, 40.3 ± 1.8%, and 5.8 ± 1.0% at 50 ㎍/mL, respectively). Compared to the basal level, oxidative damage significantly increased the mRNA levels of H2AX by 127.0 ± 8.0%. However, PR, RB, PS, and SB inhibited the expression of H2AX at mRNA level (92.6 ± 6.0%, 120.0 ± 7.6%, 68.1 ± 4.1%, and 59.4 ± 2.6% at 50 ㎍/mL, respectively). Further, oxidative damage-induced NIH/3T3 cells exhibited upregulation in the H2AX mRNA levels by 2.17 ± 0.08-fold, compared to that in the untreated group, as seen by qPCR analysis. The levels of H2AX mRNA were downregulated by PR, RB, PS, and SB treatments by 1.23 ± 0.06-fold, 1.90 ± 0.08-fold, 0.98 ± 0.04-fold, and 0.89 ± 0.03-fold, respectively at 50 ㎍/mL. The effect of antioxidant activities on various radicals in addition to their inhibitory effects on oxidative DNA damage indicates the significant potential it has towards positively impacting human health. These bioactivities were related to flavonoids and phenolic compounds, as many studies have reported a direct or indirect correlation between these compounds (Jang and Park, 2018; Mustafa et al., 2010). SB showed the highest inhibitory activity with respect to expression of γ-H2AX and p53 at protein and mRNA levels. The antioxidant activity of kazinol A and kazinol E from the root bark of B. kazinoki (Lee et al., 1997) has previously been reported. In this study, the stem bark of B. kazinoki Siebold showed the highest inhibitory activity against oxidative stress at the cellular level. Based on our study we identified SB as having a high antioxidant value, and therefore suggest that it can be used in pharmaceutical, cosmetics and food additives to alleviate and treat various diseases. Additionally, these promising results warrant further investigation into additional beneficial biological properties of this extract.
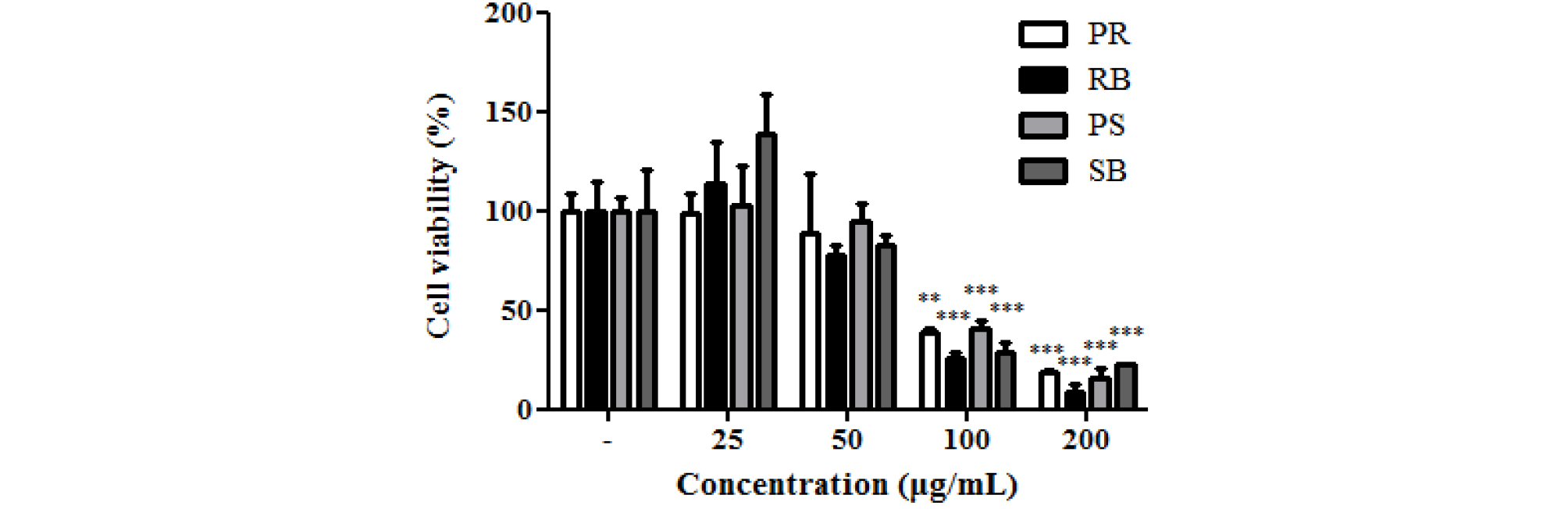
Fig. 3.
Cell viability of different parts from Broussonetia kazinoki Siebold in NIH/3T3 cells. Values are expressed as the means ± SD of three independent experiments. *p < 0.05, **p < 0.01, ***p < 0.001 as compared to non-treated group. PR; peeled root, RB; root bark, PS; peeled stem, and SB; stem bark.
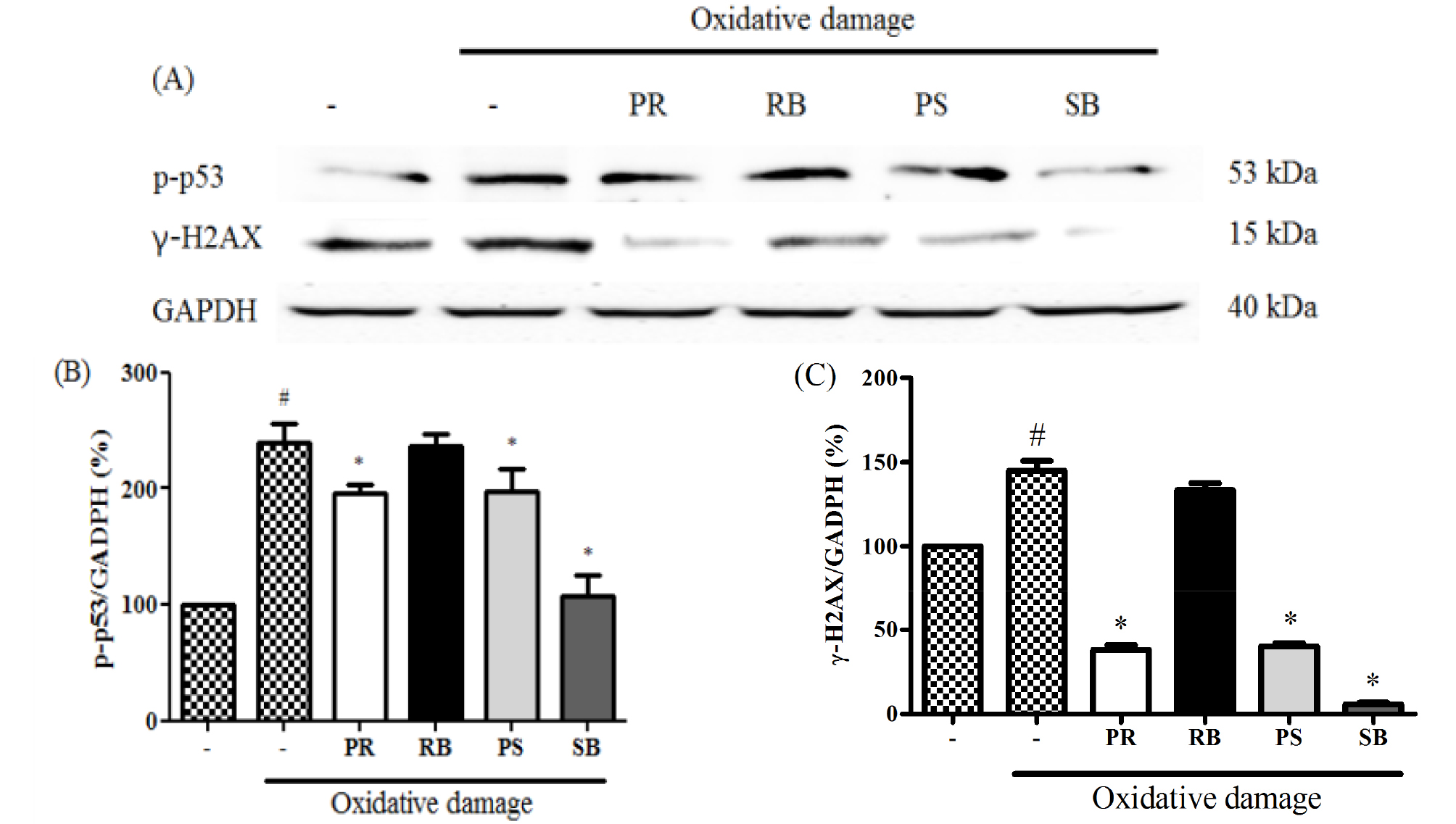
Fig. 4.
The inhibitory effect of different parts from Broussonetia kazinoki Siebold on the expression of p-p53, γ-H2AX (A), p-p53 (B), and γ-H2AX (C) normalization graphs in NIH/3T3 cells. Relative ratio was quantified after normalization to GAPDH. Values are expressed as the means ± SD of three independent experiments. *P < 0.05 vs. oxidative damage-induced group; #P < 0.05 vs. untreated group. PR; peeled root, RB; root bark, PS; peeled stem, and SB; stem bark. Oxidative damage; 150 μM FeCl2 + 500μM H2O2.
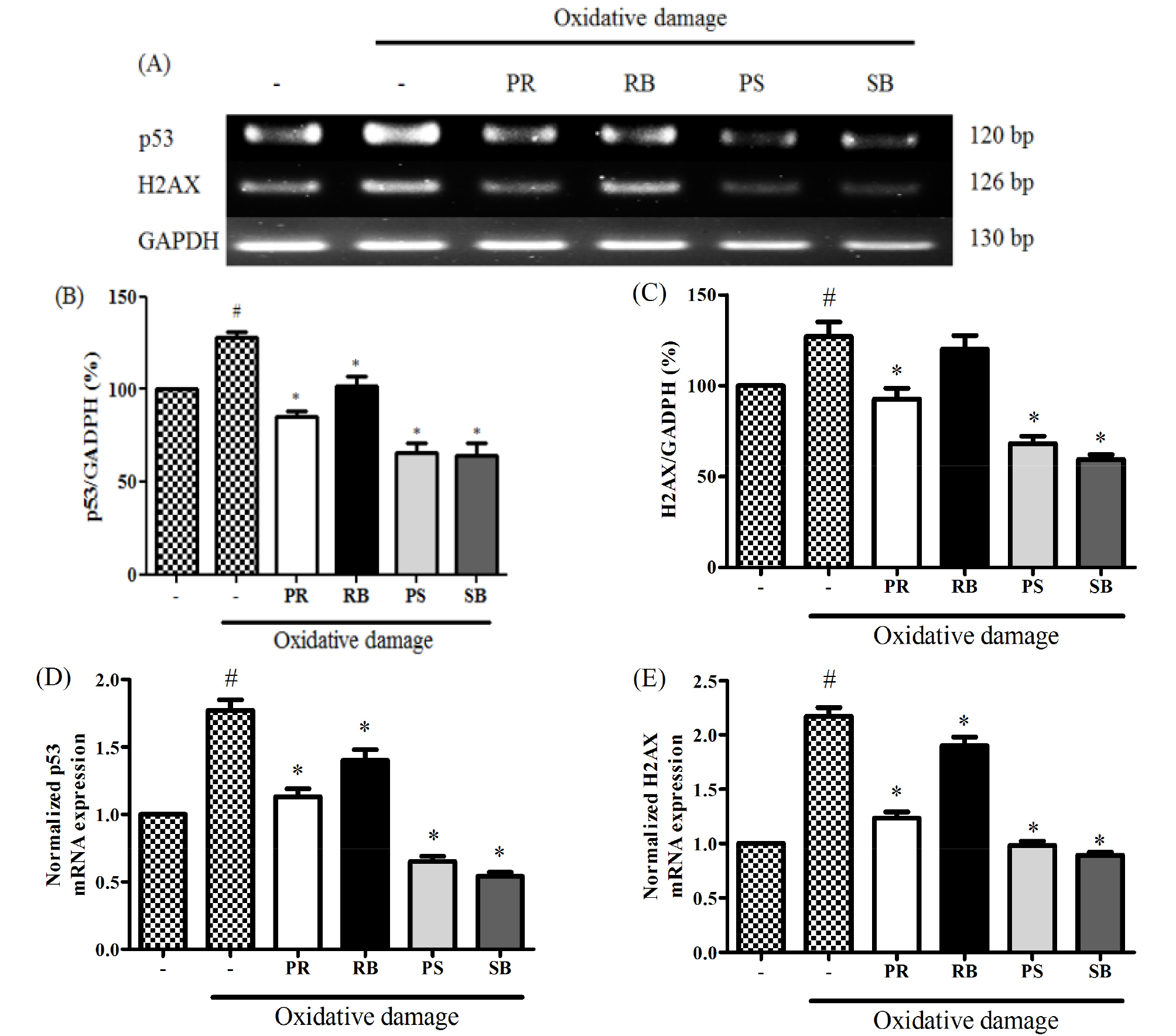
Fig. 5.
The inhibitory effect of different parts from Broussonetia kazinoki Siebold on the expression of p53, H2AX (A), p53 (B), H2AX (C) normalization graphs, qPCR analysis of p53 (D), H2AX (E) in NIH/3T3 cells. Relative ratio was quantified after normalization to GAPDH. Values are expressed as the means ± SD of three independent experiments. *P < 0.05 vs. oxidative damage-induced group; #P < 0.05 vs. untreated group. PR; peeled root, RB; root bark, PS; peeled stem, and SB; stem bark. Oxidative damage; 150 μM FeCl2 + 500μM H2O2.