Introduction
Materials and methods
Materials
Sample preparation
Cell culture and treatment
Contents of total phenol and flavonoid
DPPH and ABTS radical scavenging assay
MTT assay
Flowcytometric analysis
Western blot analysis
Statistical analysis
Results and Discussion
Antioxidative activities of AT-L
AT-L reduce the cell viability in human cancer cells
Flowcytometric analysis of AT-L in SW480 cells
Inhibitory effect of AT-L on cyclin D1 and β-catenin expression in SW480 cells
Introduction
Reactive oxygen species that occur during the breathing process in the human body are variously present, such as singlet oxygen (1O2), superoxide radical (O2-), hydrogen peroxide (H2O2), and hydroxyl radical (OH-). ROS has been known as an inducer of oxidative stress involving damage of cell matrices such as lipids, proteins, and DNA, which is associated with human diseases such as chronic inflammation and various cancers (Halliwell et al., 1992; Jin et al., 2016; Lee et al., 2014). For this reason, the evaluation of antioxidant phytochemicals constituents in natural plant extracts has received attention for their important role in the prevention of human diseases (Haq et al., 2019; Nho et al., 2018).
Acer tegmentosum Maxim (AT), a member of the family Aceraceae, is a type of deciduous tree that grows in Korea, Russia, and China. AT has been traditionally used to anticancer, leukemia, diabetes mellitus, and liver cirrhosis (Choi and Hyun, 2020; Hur et al., 2007; Kim et al., 2008; Kim et al., 2015; Yu et al., 2010). Also, AT has reported to contains steroids (β-sitosterol, β-sitosterol-3-O-β-D-glucoside and epifriedelinol) and phenol compounds such as terpenoid, salidroside, tyrosol, 6'-O-galloylsalidroside, quercetin, isoquercetin, morin-3-O-α-L-lyxoside, fraxin, cleomiscosin A, scopoletin, (+)-catechin and (-)-epicatechin (Hong et al., 2007; Kim et al., 2012; Kwon et al., 2011; Park et al., 2006; Park et al., 2016; Song et al., 2014). There are many researches on the branch and bark of AT, but little is known about the leaves of AT. Therefore, in this study, we demonstrate the antioxidative and anticancer effect of the leaves extracts of AT in human cancer cells and elucidate the mechanism of cell cycle arrest.
Materials and methods
Materials
(1,1-Diphenyl-2-picrylhydrazyl) (DPPH), 2,2'-azino-bis(3-ethylbenzothiazoline-6-sulfonic acid) (ABTS) and 3-(4,5-dimethylthiazol-2-yl)-2,5-diphenyltetrazolium bromide (MTT) were purchased from Sigma-Aldrich (St. Louis, MO, USA). Dulbecco’s Modified Eagle medium (DMEM)/F-12 1:1 Modified medium (DMEM/F-12) was purchased from Lonza (Walkersville, MD, USA). Antibodies against cyclin D1, β-catenin and β-actin were purchased from Cell Signaling (Beverly, MA, USA).
Sample preparation
The leaves of AT (AT-L, specimen number: FMCAt-201909-001) were collected in 2019 from Bonghwa-gun, Gyeongsangbuk-do, Korea, and formally identified by Hyun Jun Kim as a researcher of Forest Medicinal Resources Research Center, Korea. Twenty gram of the AT-L was extracted with 400 mL of 70% ethanol at the room temperature for 2 days (48 hours). After 48 hours, the extracts were filtered, concentrated a vacuum evaporator, and freeze-dried. The freeze-dried extracts were kept in a refrigerator until use.
Cell culture and treatment
Human cancer cells were purchased American Type Culture Collection (ATCC, Virginia, USA) and grown in Dulbecco's Modified Eagle (Lonza, Walkersville, MD, USA) supplemented with 10% fetal bovine serum (FBS), penicillin (100 units/mL) and streptomycin (100 ㎍/mL). The cells were incubated at 37℃ under a humidified atmosphere of 5% CO2. The extracts from AT were dissolved in dimethyl sulfoxide (DMSO) and treated to cells. DMSO was used as a vehicle.
Contents of total phenol and flavonoid
The total phenolic contents were determined by using the Folin-ciocalteu method with some modification (Singleton et al., 1999). To 10 μL of AT, 200 μL of 2% Na2CO3 and 10 μL of 50% Folin-ciocalteu reagent were added. The mixture was incubated at room temperature for 90 minutes. After 90 minutes, the absorbance was measured at 760 ㎚. Quantification was performed based on the standard curve of gallic acid. The flavonoid contents were determined by a colorimetric method with minor modification (Woisky and Salatino, 1998). To 20 μL of AT, 80 μL of distilled water and 6 μL of 5% NaNO2 were added. The mixture was incubated at room temperature for 5 minutes. After 5 minutes, 10 μL of 10% Aluminum nitrate was added. After 6 minutes, 40 μL of 1N NaOH was added. The mixture was incubated at room temperature for 11 minutes. After 11 minutes, the absorbance was measured at 415 ㎚. Quantification was performed based on the standard curve of rutin.
DPPH and ABTS radical scavenging assay
DPPH radical scavenging assay was carried out according to the methods of Blois (Blois, 1958). Briefly, 90 μL DPPH ethanol solution and 10 μL of the extracts were mixed and then incubated at 37℃ for 30 minutes. After 30 minutes, the absorbance was measured at 515 ㎚ (Perkin Elmer, Waltham, MA, USA). ABTS radical scavenging assay was carried out according to the methods of Re et al. (1999). Briefly, 90 μL ABTS solution and 10 μL of the extracts were mixed and then incubated at room temperature for 10 minutes. After 10 minutes, the absorbance was measured at 732 ㎚ (Perkin Elmer, Waltham, MA, USA).
MTT assay
Human cancer cell viability was estimated by determining mitochondrial reductase function with the MTT assay. Briefly, cancer cells (3 × 104 cells/well) were cultured in 12-well plate and incubated for 24 hours. The cancer cells were treated with AT-L at the indicated concentrations for 24 hours. Then, the cells were cultured with 200 μL of 1 ㎎/mL MTT solution for an additional 2 hours. The resulting crystals were dissolved in DMSO. The formazan synthesized was dissolved in DMSO and the optical density was measured at 570 ㎚ using UV/Visible spectrophotometer (Perkin Elmer, Waltham, MA, USA).
Flowcytometric analysis
Human colorectal cancer cells (3 × 104 cells/well) were cultured in 12-well plate for overnight. The cells were pretreated with AT-L at the indicated concentrations (0, 12.5, 25 and 50 ㎍/mL) for 24 hours. After 24 hours, cells were harvested in ice-cold 1 X phosphate-buffered saline (PBS). Human colorectal cancer cells (SW480 cells) were prepared using a cell cycle kit (Beckman coulter, Brea, CA, USA) according to the manufacturer's protocol for flow cytometer.
Western blot analysis
After treatment with AT-L, SW480 cells were harvested in ice-cold 1×PBS and lysed in radioimmunoprecipitation assay (RIPA) buffer (Boston Bio Products, Ashland, MA, USA) supplemented with phosphatase inhibitor cocktail and protease inhibitor cocktail, and centrifuged at 15,000 rpm for 10 minutes at 4℃. The protein content of the cell lysates was then determined using the bicinchoninic acid (BCA). Protein in each sample was resolved by 10% sodium dodecyl sulfate polyacrylamide gel electrophoresis (SDS-PAGE), transferred to the nitrocellulose membranes (Bio-Rad, CA, USA). The membranes were blocked for non-specific binding with 5% skim milk in Tris-buffered saline containing 0.05% Tween 20 (TBS-T) for 1 hour at room temperature. The membrane was incubated with diluted primary antibodies for proper times and then washed three times with TBS-T and further incubated with secondary antibody for 1 hour at room temperature. The chemiluminescence was detected with ECL Western blotting substrate and visualized in the Chemi Doc MP Imaging system (Bio-rad, CA, USA).
Statistical analysis
Data are expressed as mean ± standard deviation (SD). Each experiment was repeated at least three times. Statistical analysis was performed with the Students unpaired t-test, with statistical significance set at *, P < 0.05.
Results and Discussion
Antioxidative activities of AT-L
Phytochemistry, such as flavonoid and polyphenol, has been reported to effects on oxidative removal, gene control, and immune system stimulation in vivo (Luthria et al., 2105). As shown in Fig. 1A, the total phenolic contents of AT-L were 10.74 gallic acid equivalents (GAE) ㎍/mL and total flavonoids contents of AT-L were 5.68 rutin equivalents (RE) ㎍/mL, respectively. Antioxidant activities can be defined as inhibition of oxidative damage of organic molecules including lipids, proteins, DNA, and other molecules (Huang et al., 2005). Antioxidant activities of AT-L were evaluated using DPPH and ABTS radical scavenging assay. Scavenging of DPPH and ABTS radicals has been used as the basis of a common antioxidant assay. In ABTS radical scavenging activity (Fig. 1B), AT-L and ascorbic acid scavenged ABTS radical by 99.6% and 69.78 at 8 ㎍/mL, respectively. Especially, the AT-L measured the highest ABTS radical scavenging activity, which is higher than ascorbic acid. In DPPH radical scavenging activity (Fig. 1C), AT-L scavenged DPPH radical by 43.95% at 12.5 ㎍/mL and 67.83% at 25 ㎍/mL, respectively. Ascorbic acid scavenged DPPH radical by 45.26% at 12.5 ㎍/mL and 75.39% at 25 ㎍/mL, respectively. The results show that AT-L can be used as antioxidative agents.
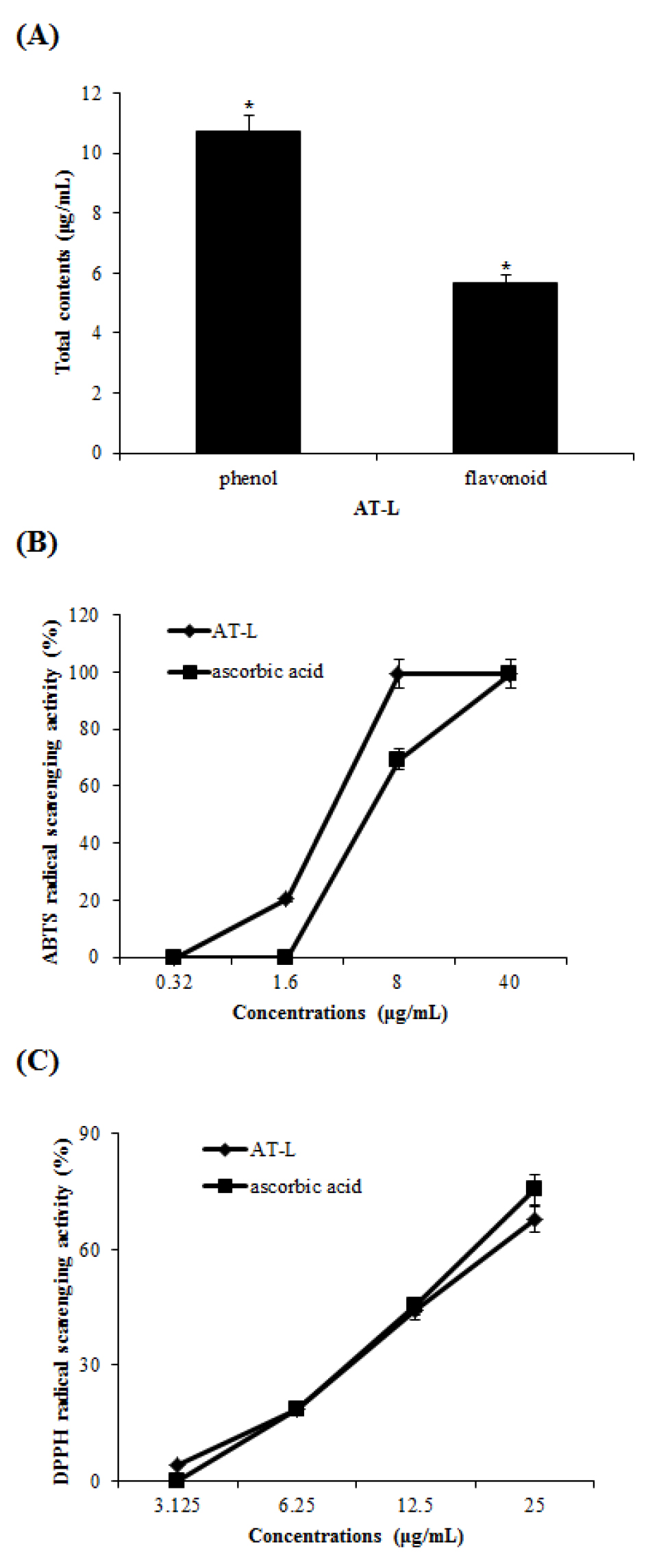
Fig. 1
(A) The total phenol and flavonoid contents of AT-L. (B and C) ABTS and DPPH radical scavenging activity of the AT-L. Ascorbic acid was used as a positive control. Comparing with the same concentration of ascorbic acid, respectively. All data are from one experiment, representing three independent experiments.
AT-L reduce the cell viability in human cancer cells
To investigate if AT-L affects the proliferation of human cancer cells, colorectal cancer (SW480), prostate cancer (PC-3), breast cancer (MCF-7), pancreatic cancer (AsPC-1), lung cancer (A549) and liver cancer (HepG2) cells were treated with the different concentrations of AT-L for 24 hours and the cell proliferation was measured. As shown in Fig. 2A, SW480 cells treated with 0, 25, 50, and 100 ㎍/mL of AT-L reduced cell growth by 29 %, 59 %, and 78 % in 24 hours, respectively. PC-3 cells treated with 0, 25, 50, and 100 ㎍/mL of AT-L reduced the cell growth by 20 %, 33 %, and 68 % in 24 hours, respectively (Fig. 2B). AsPC-1 cells treated with 0, 25, 50, and 100 ㎍/mL of AT-L reduced the cell growth by 18 %, 52 %, and 68 % in 24 hours, respectively (Fig. 2C). A549 cells treated with 0, 25, 50, and 100 ㎍/mL of AT-L reduced cell growth by 30 %, 58 %, and 75 % in 24 hours, respectively (Fig. 2D). MCF-7 cells treated with 0, 25, 50, and 100 ㎍/mL of AT-L reduced the cell growth by 3 %, 15 %, and 53 % in 24 hours, respectively (Fig. 2E) and HepG2 cells treated with 0, 25, 50, and 100 ㎍/mL of AT-L reduced the cell growth by 12 %, 44 %, and 67 % in 24 hours, respectively (Fig. 2F). Among human cancer cells, AT-L showed the highest inhibition of cell proliferation in SW480 cells. The results indicate that AT-L suppressed cell growth of human cancer cells in a dose-dependent manner.
Flowcytometric analysis of AT-L in SW480 cells
Flowcytometry can efficiently sort cells and sensitively detect based on fluorescent signals integrated into cellular markers of proteins or DNA. It has been widely applied to assess cell division, apoptosis, and to isolate cells including stem cells (Gupta et al., 2011). Flow cytometric evaluation of PI fluorescence allows the quantification of both stages of the cell cycle and apoptosis. The amount of sub-G0/G1 DNA increases as the degree of fragmentation increases due to an apoptotic insult (Pozarowski and Darzynkiewicz, 2004; Wlodkowic et al., 2009). We investigated whether AT-L inhibits the SW480 cell cycle. The SW480 cells treated with 0, 12.5, 25, and 50 ㎍/mL of AT-L showed the cell cycle sub-G1, G0/G1, S, and G2/M peak (Fig. 3). The results indicate that AT-L arrested the cell cycle of SW480 cells in a dose-dependent manner.
Inhibitory effect of AT-L on cyclin D1 and β-catenin expression in SW480 cells
Wnt/β-catenin signaling is an evolutionarily conserved and multifaceted pathway that is known to be involved in tissue homeostasis, embryonic development, and a wide variety of human diseases. Aberrant activation of this pathway gives rise to the accumulation of β-catenin in the nucleus and promotes the transcription of many oncogenes such as Cyclin D1 and c-Myc (Kang et al., 2020; Shang et al., 2017). As one of the proto-oncogene, cyclin D1 is an important regulator of G1 to S progression by forming an active complex with cyclin-depending kinase (CDK) 4 and 6 in many different cell types. Cyclin D1 and β-catenin have been reported to be key for the progression and development of several cancers, such as lung, breast, bladder, and esophagus (Alao, 2007; Knudsen et al., 2006; Park et al., 2017).
To test if AT-L affects cyclin D1 and β-catenin expression, we treated SW480 cells with 0, 12.5, 25, and 50 ㎍/mL of AT-L for 24 hours. As shown in Fig. 4A, AT-L treatment down-regulated cyclin D1 and β-catenin expression. Next, we observed the effects of AT-L on cyclin D1 and β-catenin expression at different time points. As shown in Fig. 4B cyclin D1 expression begins to decrease at 10 hours and β-catenin expression begins to decrease at 6 hours in SW480 cells. These results showed that AT-L decreased cyclin D1 and β-catenin expression in SW480 cells, which indicates that cyclin D1 and β-catenin may be a potential molecular target for the induction of cell cycle arrest by AT-L.
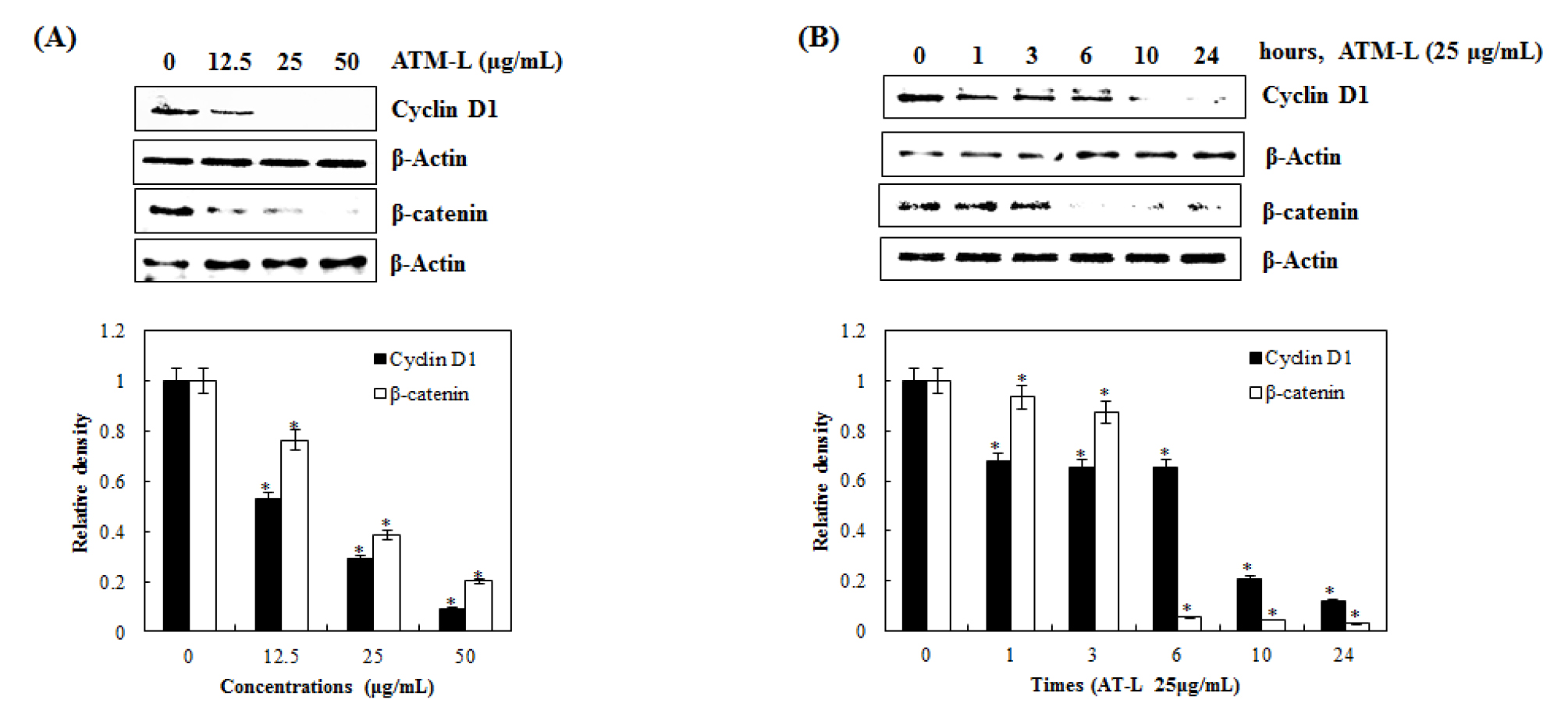