Introduction
Materials and Methods
Plant materials
Lesion development assay using leaves of B. rapa and inoculation of B. cinerea isolates
Measuring days to flowering and plant height
Counting numbers of aphids and thrips
Statistical analysis
Results and Discussion
Parental lines and RIL population behaves differently against five B. cinerea isolates
Response to insects: Aphids and thrips
Vegetative growth estimated by measuring days to flowering and plant height
Comparative analysis among various phenotypic traits
Introduction
The species Brassica rapa is one of the most valuable vegetable crops worldwide. It is an important vegetable, oilseed, and fodder crop. B. rapa (2n = 20) is one of diploid species in genus Brassica possessing subgenome A while other diploid species B. nigra (2n = 16) and B. oleracea (2n = 18) possess subgenomes B and C, respectively (FitzJohn et al., 2007; Mason and Snowdon, 2016). Cultivated varieties of B. rapa exhibit diverse developmental and morphological appearances, including leafy vegetables, turnips, and oil types that can be categorized differently depending on which organs are consumed (Guo et al., 2014; Zhao et al., 2005). Especially, many crops of B. rapa, including heading Chinese cabbage, nonheading Chinese cabbage, turnip, and yellow mustard, show obvious differences in leaf morphology.
Botrytis cinerea causes significant economic losses for Brassica crops worldwide, in addition to other multiple pathogens such as clubroot fungus (Plasmodiophora brassicae), soft rot decay bacteria (Pectobacterium carotovorum), turnip mosaic virus (TuMV), Meloidogyne root knot nematodes, and so on. B. cinerea is a generalist necrotrophic pathogen that causes lesions for nearly all harvestable parts of Brassica plants, including leaves, stems, florets, and pods (Bolton et al., 2006; Williamson et al., 2007). Although there is a great interest in breeding for broad spectrum resistance of Brassica crop species to B. cinerea, such breeding is complicated due to the fact that B. cinerea is a highly polymorphic pathogen possessing high levels of genetic variations in virulence mechanisms (Amselem et al., 2011; Rowe and Kliebenstein, 2008; Staal et al., 2008; Staats and van Kan, 2012). Therefore, breeding effort and genetic studies about resistance to B. cinerea have typically focused on race-specific resistance and individual B. cinerea isolates. Quantitative trait loci (QTLs) controlling resistance to B. cinerea have also been previously reported (Zhang et al., 2016).
In addition to diseases caused by phytopathogens, insect herbivores are also major threats to agriculture by delaying plant growth and decreasing crop productivity. Aphid, a notorious pest for a wide range of crops worldwide, causes economic losses of B. rapa by sucking plant sap and transmitting viruses in the feeding process (Bragard et al., 2013; Howe and Jander, 2008; Powell et al., 2005). Aphid species that cause damages to Brassica genus include green peach aphid (Myzus persicae), cabbage aphid (Brevicoryne brassicae), and turnip aphid (Lipaphis erysimi) (Jones et al., 2001). All developmental stages of the crop can be attacked by aphids. Considerable damage occurs during flowering and pod formation stages (Bhatia et al., 2011; De Vos et al., 2007; Goggin, 2007). Impact of aphids on yield reduction is influenced by the growth stage of the crop during aphid infestation, duration and intensity of infestation, soil and environmental conditions, and so on. Thrips are also hazardous herbivores responsible for serious damages to a wide range of crops in many countries. Onion thrips (Thrips tabaci) and western flower thrips (Frankliniella occidentalis) are major thrip species that affect crop cultivation, causing direct plant damage. They can also act as vectors that transmit serious plant diseases during the feeding process (Diaz-Montano et al., 2011; Hogenhout et al., 2008).
Flowering time is an important developmental trait of B. rapa. It determines the transition from vegetative growth to reproductive growth that show a wide variations among B. rapa species (Bloomer and Dean, 2017; Koornneef et al., 2004; Roux et al., 2006). Flowering time is also important for the yield of crops or vegetables. Regulating the flowering time is an important goal of plant breeding. Flowering time is influenced by both internal and external factors including day-length, light intensity, and temperature (Lou et al., 2007; Li et al., 2015). Many Brassica species requires vernalization for flowering. Vernalization refers to acquisition of the ability to flower by prolonged exposure to cold temperatures (Amasino, 1996; Chouard, 1960; Shea et al., 2017).
Responses of 113 recombinant inbred lines (RILs) derived from crosses between two breeding lines, IMB211 and R500 (Iniguez-Luy et al., 2009), to five isolates of B. cinerea were evaluated. Flowering time, plant height, and numbers of aphids and thrips inhabited on each plant genotype during the flowering stage were measured for 113 RILs. Since developing a breeding line with reliable and durable resistance to B. cinerea in B. rapa would be a highly complicated and difficult task to accomplish due to numbers of QTLs controlling phenotypic outcomes and their genotype specific interactions, it is important to determine the target B. cinerea isolate in resistance breeding program. To understand the nature of genetic factors and the relationship among these phenotypes, correlation analysis was performed. Significant correlation between certain combinations of phenotypic measurements was found. This will be further discussed later in this paper.
Materials and Methods
Plant materials
Two parental lines of B. rapa, R500 (male) and IMB211 (female), and their resulting recombinant inbred lines (RILs, n = 113) (Williams and Hill, 1986; Iniguez-Luy et al., 2009) were used in this study. Both parental lines and 133 RILs were cultivated in the University of California, Davis greenhouses under a 12 h photoperiod using a day/night temperature set at 25/18℃ with relative humidity of 70% (Fig. 1). Plants were grown in 15 ㎝-diameter plastic pots filled with standard potting soil (Sunshine Mix #1; Sun Gro Horticulture) with fertilizer. To prevent cross pollination, all plants were bagged with mesh pollination bag during flowering. Watering was conducted once a day. Watering time was gradually reduced in about two weeks for seed maturation. Seeds were harvested when the majority of seed pods were dry. They were stored separately.
Lesion development assay using leaves of B. rapa and inoculation of B. cinerea isolates
Five B. cinerea isolates (Ausubel, Davis Navel, Pepper, Katie Tomato, and UK Razz) were used in this study. Information about these isolates has been described in previous reports (Denby et al., 2004; Kliebenstein et al., 2005). All isolates were maintained as conidial suspensions in 30% glycerol at -80℃ for long time storage. Conidial suspensions were swabbed on freshly prepared potato dextrose agar (PDA, Gibco/Invitrogen, Carlsbad, CA, USA) medium and cultured at room temperature. Spores used for infecting B. rapa leaves were collected using previously described method (Rowe and Kliebenstein, 2008).
The detached leaf assay has been widely utilized to assess plant resistance to necrotrophic fungi (Zhang et al., 2016). It was used in this study. Each seed was sown into a 5 ㎝-diameter plastic pot filled with standard potting soil (Sunshine Mix #1; Sun Gro Horticulture) and water. All pots were cultivated at 25 ± 1℃ under a 16 h photoperiod for 10 days after conducting vernalization treatment for 3 days at 4℃. The first fully developed leaves were detached and placed on 1.2% phytoagar in large plastic trays and inoculated with 4 µL droplets of B. cinerea spore suspensions (10 spores/µL) in 50% filtered grape juice (Santa Cruz Organics, CA, USA) at room temperature in light condition. Control leaves (mock) were inoculated with 4 µL droplets of 50% filtered grape juice without spores. All inoculated leaves were incubated at room temperature with light illumination. Four independent biological replications were used for each isolate/genotype pair. Lesion development was monitored every 8-12 h. The fungal lesion size for each isolate was digitally measured and determined at 72 h post inoculation and statistically analyzed.
Trypan blue staining was performed to visualize the growth of B. cinerea mycelium and plant cell death caused by fungal infection. Trypan blue staining of leaves was performed at 12 h post inoculation as previously described (van Wees, 2008). Briefly, inoculated leaves were transferred into a 50 mL plastic tube containing 2.5 ㎎/mL trypan blue-lactophenol solution diluted in ethanol (1 :2 v/v). These sample tubes were left in staining solution at room temperature for about 12 h after boiling at 100℃ for 1 min. The staining solution was removed and leaf tissues were treated with chloral hydrate solution for 6 h for de-staining. After de-staining, leaf tissues were treated with 70% glycerol. High-resolution digital photos were taken for entire leaves and each lesion for visualization.
Measuring days to flowering and plant height
Both parental lines and 133 RILs treated for vernalization (4℃ for 5 days) were transplanted in 15 ㎝-diameter plastic pot filled with standard potting soil (Sunshine Mix #1; Sun Gro Horticulture) with fertilizer. Plants were cultivated under a 12 h photoperiod using a day/night temperature set at 25/18℃ with a relative humidity of 70%. Each plant was covered with a pollination bag at one week after transplanting. Days to flowering were determined based on the day when the first flower fully bloomed. Plant height was measured at the harvesting point. Three independent plants were used for measuring both days to flowering and plant height.
Counting numbers of aphids and thrips
Numbers of aphids or thrips on each plant were determined based on the number of insects at the time when seeds were harvested. Insects remained in pollination bags at the harvesting point (two weeks after stopping irrigation of all pots) were collected and counted thoroughly. Three independent biological replications were used for each insect/genotype pair.
Statistical analysis
Data were statistically analyzed with SigmaPlot program (ver. 12, SPSS Inc., USA). Coefficients of relationship between lines and strains for each estimation were calculated and fitted using linear regression equation.
Results and Discussion
Parental lines and RIL population behaves differently against five B. cinerea isolates
Detached leaf assay using trypan blue staining of B. cinerea lesions on B. rapa was conducted for parental lines and 113 RILs. Lesion size observed in detached leaf assay using five different B. cinerea isolates showed variations among them (Fig. 2), suggesting that genetic factors controlling resistance or tolerance against each isolates varied in this population. Lesion development in B. rapa caused by B. cinerea inoculation was visualized by trypan blue staining (Fig. 2). Trypan blue staining of detached leaves allowed monitoring of the growth of B. cinerea mycelium and plant cell death caused by fungal infection.
Development of lesion and the spread of mycelium growth of two parental lines were compared. Relatively smaller lesions and less spread of mycelium growth were detected for R500 compared to another parental line IMB211 in most cases. This was more obvious when leaves were inoculated with B. cinerea isolates, Pepper, Ausubel, or Davis Navel, suggesting that R500 tended to be more tolerant against these three isolates than IMB211. Although several RIL lines showing smaller lesion sizes than those of the tolerant parental line R500 (Fig. 3), no RIL with tolerance higher than R500 against all tested B. cinerea isolates was found. According to lesion diameter measurements, two parental lines showed no significant difference in response to the following two B. cinerea isolates: Katie Tomato and UK Razz. In the case of UK Razz isolate, it caused relatively small lesion size compared to lesions caused by other isolates in both parental lines and in almost all RILs. This implies that both parental lines are tolerant. This isolate and/or UK Razz possessed the weakest virulence in these germplasms. Although both parental lines showed moderate susceptibility against isolate Katie Tomato, these RILs showed variations, suggesting that genetic factors involved in defense against isolate Katie Tomato might be present in these germplasms. Outcomes of these genetic factors appeared to vary depending on their distribution in this population.
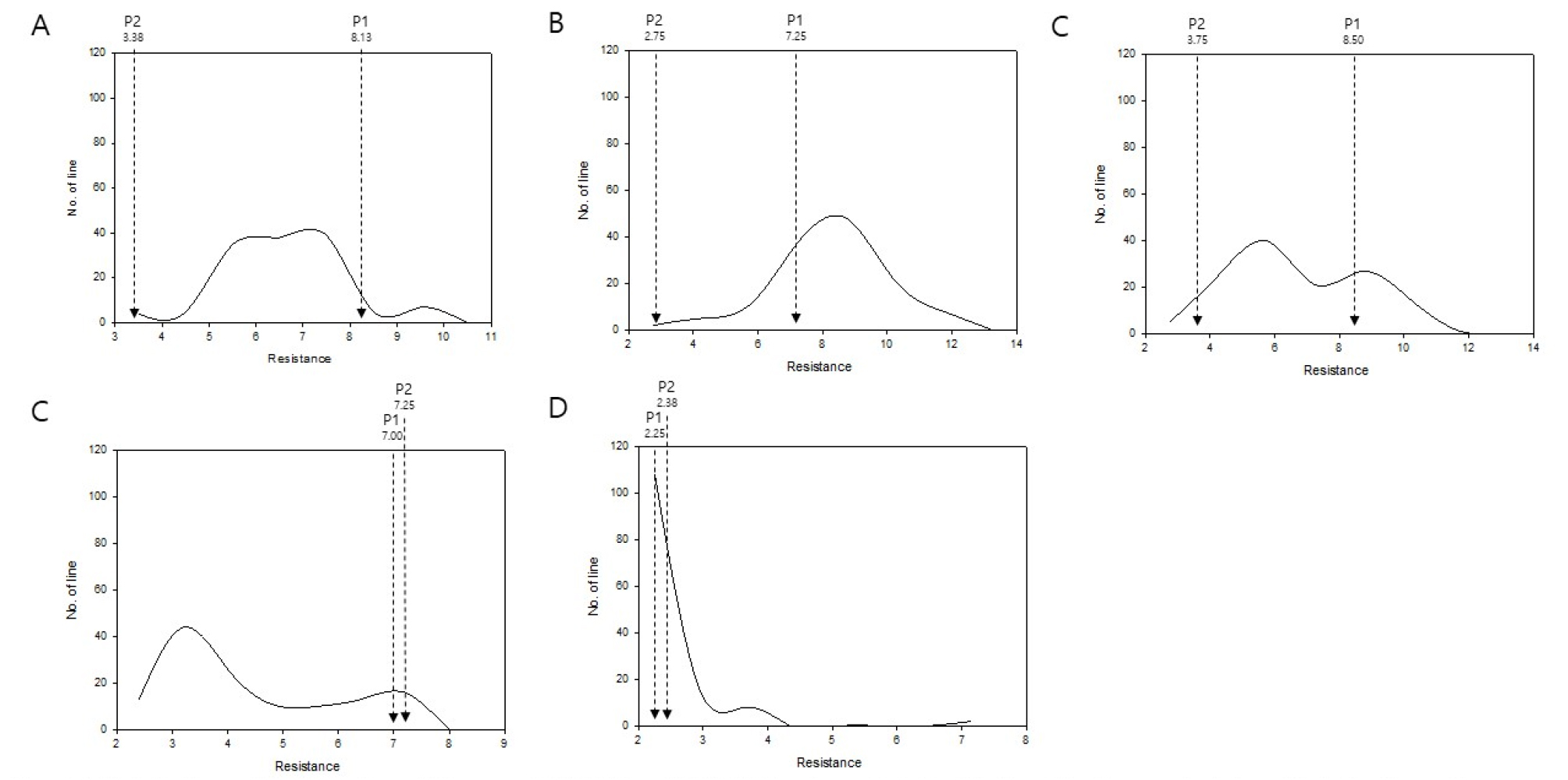
Fig. 3
Distribution of lesion size of B. rapa IMB211 × R500 RILs inoculated with five B. cinerea isolates. Distribution of mean lesion size (mm) on leaves of B. rapa IMB211 × R500 RILs measured at 72 h after inoculation with five Botrytis isolates: (A) Ausubel, (B) Davis navel, (C) Katie tomato, (D) Pepper, and (E) UK Razz. P1: IMB211, P2: R500. Arrow indicates mean lesion size on IMB211 (P1) and R500 (P2).
Although several RILs showed smaller lesion sizes compared to R500 against specific isolate(s), there was not a single RIL showing smaller lesion size compared to both of their parental lines based on data for all five different B. cinerea isolates. Considering numbers of QTLs controlling B. cinerea resistance and their genotype are specific in nature, it is obvious that developing a breeding line with reliable and durable resistance to multiple B. cinerea isolates in B. rapa would be a highly complicated and difficult task to accomplish. These results suggest the presence of genotypic dependent interactions between B. rapa and B. cinerea isolates. This has been previously suggested by Zhang et al. (2016).
To better understand the nature of genetic factors regulating the resistance against these five B. cinerea isolates, a correlation analysis was performed. Correlation coefficients were calculated using responses of 113 RILs to these five isolates (Pepper, Ausubel, Davis Navel, Katie Tomato, and UK Razz) of B. cinerea (Table 1). Significant positive correlation (p < 0.05) was observed for several combinations of these isolates: Pepper-Ausubel, Pepper-Davis Navel, Ausubel-Davis Navel, and Davis Navel-Katie Tomato. Isolate UK Razz showed very narrow range of data distribution. It did not show significant correlation with any other isolate. Isolate Katie Tomato showed a wide range of data distribution for RILs when no clear difference between two parents was detected. It showed a positive correlation only with Davis Navel. Based on correlation analysis results, despite a genotypic dependent interaction between B. rapa and B. cinerea observed in previous studies, it can be speculated that certain parts of genetic components controlling resistance against Pepper, Ausubel, and Davis Navel isolates are overlapped. Further assays using QTL mapping focusing on genetic factors associated with the positive correlation among these isolates are needed to understand genetic factors responsible for the broad spectrum resistance of B. rapa against B. cinerea.
Table 1.
Correlation analysis between phenotypic data collected for 113 RILs in this study (values listed in this table indicate Pearson Correlation Coefficients or PCC)
Response to insects: Aphids and thrips
Numbers of aphids and thrips remained in pollination bags at the harvesting point were counted. A higher population of these insects was considered to indicate a relative susceptibility or/and vulnerability of the host plant to these insects. Both aphids and thrips were less populated in IMB211 than in R500, suggesting that IMB211 was more tolerant or less preferred by both insects. The number of aphids on each RILs were roughly distributed normally. Less numbers of aphids were detected on 11 of 113 RILs compared to those on IMB211. The number of thrips on each RIL appeared to be skewed toward tolerance or less preferred by thrips. Over 50 lines showed less numbers of thrips compared to IMB211. It was interesting to note that most of those 11 lines with less numbers of aphids also showed less numbers of thrips on them (data not shown). These results suggest that plants that are tolerant or less preferred by aphids are also efficient in repelling thrips in this study. Correlation analysis between numbers of aphid and thrips in RILs indicated a significant positive correlation (Table 1). Based on these results, it can be speculated that genetic factors responsible for aphid tolerance or repellence could be also involved in thrip tolerance or repellence. Furthermore, these results give us clue that gaining aphid tolerance might also gain thrip tolerance together.
Genetic factors affecting insect tolerance of plants are generally considered to have a great complexity. Numbers of biotic and abiotic factors can influence outcomes of plant-insect interactions. The most well-studied area in plant-insect interaction is about secondary metabolites of plants responsible for defense mechanisms, including terpenes, phenolics, nitrogen- and sulfur-containing compounds (Hopkins et al., 2009; Mitchell et al., 2016). Data presented in this study can be used as preliminary data for understanding genetic interaction factors controlling insect density or/and RIL population.
Vegetative growth estimated by measuring days to flowering and plant height
Days to flower were determined based on the day when the first flower fully bloomed. IMB211 needed 12.67 days to flowering, which was significantly shorter than R500 which needed 26.00 days to flower. There were 56 lines of 113 RILs showing earlier flowering time compared to both of their parental lines. Data were not normally distributed. They were skewed toward early flowering as shown in Fig. 4C. In general, earliness of flowering is preferable. It is an important objective of breeding Brassica species. As shown in Figs. 1 and 4D, the plant height of IMB211 (mean value: 23.33 ㎝) was significantly shorter than R500 (mean value: 66.00 ㎝). Data from RILs were normally distributed. Mean values for plant height lie in between 8 ㎝ and 53.17 ㎝. It was interesting to note that not a single RIL was taller than one of their parental line R500, although all plants including parental lines were grown together and treated evenly.
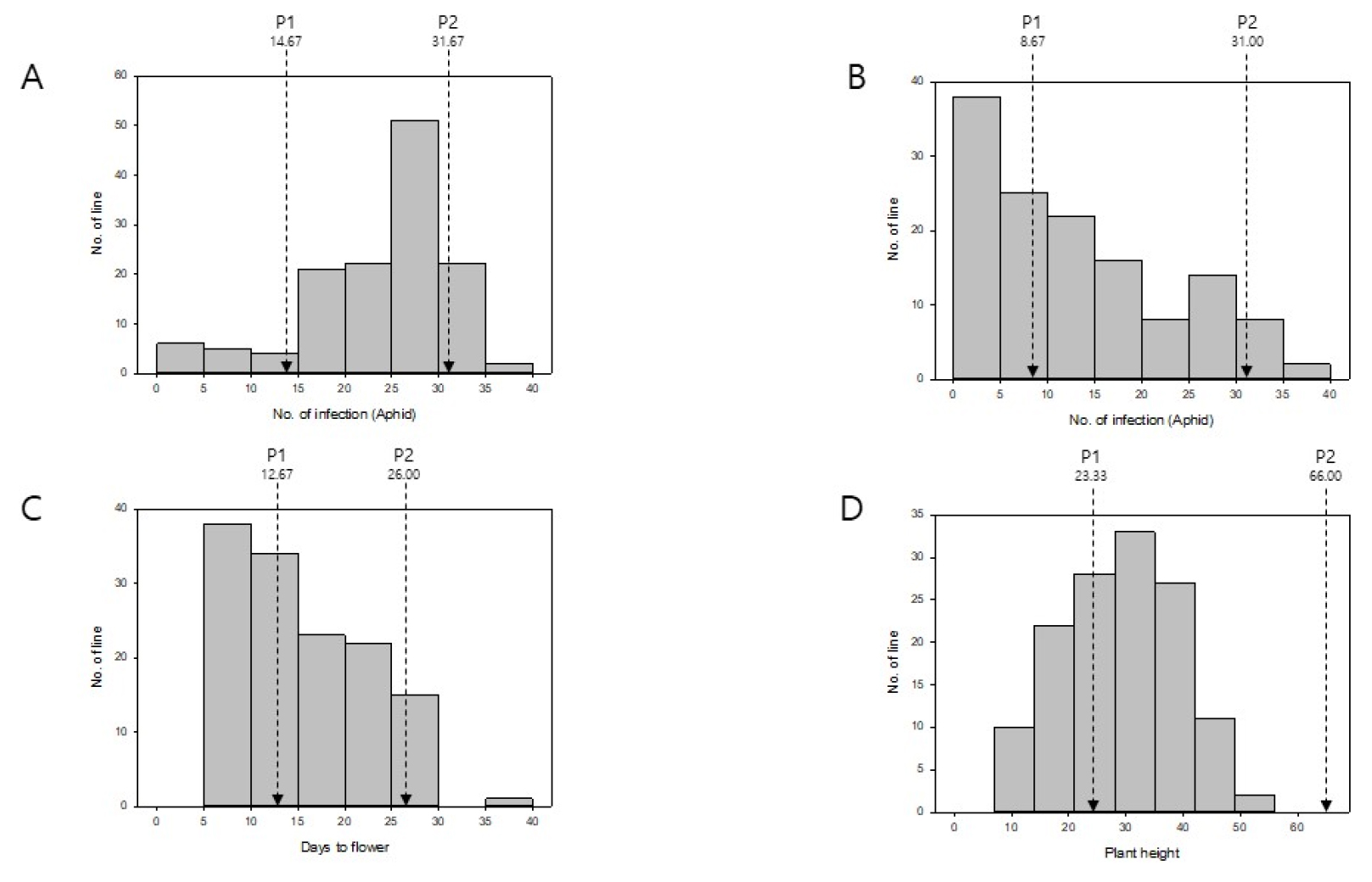
Fig. 4
Distribution of phenotypic variations of B. rapa IMB211 × R500 RILs. Four different phenotypes were measured for B. rapa IMB211 × R500 RILs. A: Number of aphid infection. B: Number of thrip infection. C: Days to flowering. D: Plant height. P1: IMB211, P2: R500. The bold arrow indicates mean value on IMB211 and the blank arrow indicates mean value on R500.
Correlation analysis between days to flowering and plant height in RIL population showed a significant negative correlation (Table 1), implying that plants with less days to flowering show certain levels of tendency to be taller. Vegetative growth represents plant growth from germination to flowering, which mostly covers photosynthesis and accumulating resources required for flowering and reproduction. Numerous factors are involved in various forms of vegetative growth. There are enormous levels of complexity among them. Those factors include multiple genes, transcriptional factors regulating those genes, hormonal regulation, and biotical and abiotical environmental effects. The relationship between plant height and flowering traits has been well studied in Arabidopsis and maize, and believed to be closely correlated (Song et al., 2012; Vanous et al., 2018). These two traits significantly affecting plant yield are known to be highly heritable and strongly influenced by climatic and day-length adaptation (Kim et al., 2016; Song et al., 2012; Vanous et al., 2018). Moreover, both traits are commonly affected by a plant hormone gibberellin. In this study, we provided brief measurements for days to flowering and plant height and detected a tendency that plants with less days to flowering appears to be taller, which could be further explored and utilized in Brassica breeding. Further investigation is required to better understand the vegetative growth of this RIL population.
Comparative analysis among various phenotypic traits
In this study, various phenotypes of recombinant inbred lines (RILs) of B. rapa were investigated, including their responses to five different pathogenic B. cinerea isolates, responses to aphids and thrips during flowering stages, and vegetative growth including days to flowering and plant heights. Data obtained for these phenotypes were used for correlation analysis (Table 1). Although high levels of correlation were not detected between phenotypes assessed in this study, implying a complex and independent manner of each genetic component controlling each phenotype, statistically significant correlation was detected for several combinations. Lesion size caused by five B. cinerea isolates and number of insects remained on plants did not provide meaningful conclusion, although a significant negative correlation was detected for several combinations. It was observed that IMB211 that showed weaker fungal tolerance to B. cinerea was more efficient in repelling both insects than another parental line R500. It was interesting to note that plant height measurements showed significant correlations with numbers of aphid and thrips remained on plants, with taller plants showing certain levels of tendency to have more insects on them. Based on this correlation analysis, it could be speculated that shorter plants might have more tolerance or repellence against aphid and thrips. Since all phenotypic data obtained in this study were parts of complex traits, it was difficult to draw a concise conclusion.
Negative correlation between vegetative growth and defense has been discussed previously, which generally explained as growth-defense tradeoffs resulted by to the plant’s allocation of limited resources (Karasov et al., 2017; Züst and Agrawal, 2017). The resource restrictions for protective compounds and associated morphological structures are thought to demand prioritization towards either growth or defense, depending on external and internal factors (Campos et al., 2016). These growth–defense tradeoffs appears to have profound implications in agriculture and natural ecosystems, since both growth and defense processes are vital for plant survival, reproduction, and, ultimately, plant fitness (Huot et al., 2014). Data obtained from our study partially supports these growth-defense tradeoffs. Most obviously, significant negative correlation between repellence against aphids or thrips and plant height is observed. The relations between defense against B. cinerea and vegetative growth is less conclusive based on our study. Overall data presented in this study could be used to assist further genetic and ecological studies and to support breeding efforts to obtain Botritis and insect resistance for B. rapa.