Introduction
Materials and Methods
Reagents
Preparation of ZP
Cell culture
MTT assay
Cytokine assay
PGE2 assay
Measurement of nitrite (NO) concentration
Western blot analysis
Luciferase reporter gene assay
Caspase-1 activity assay
Statistical analysis
Results
ZP inhibits production of TNF-α and IL-6 in LPS-induced RAW264.7 cells
ZP inhibits production of PGE2 and expression of COX-2 in LPS-induced RAW264.7 cells
ZP inhibits production of NO and expression of iNOS in LPS-induced RAW264.7 cells
ZP inhibits NF-κB activation in LPS-induced RAW264.7 cells
ZP inhibits caspase-1 activation in LPS-induced RAW264.7 cells
Discussion
Introduction
Inflammatory response is a process that involves the action of multiple factors within a complex network (Hawiger, 2001). Although the inflammatory reaction is considered to be an important response for the host defense against infection, bacteria, or bacterial products, prolonged inflammation can lead to various chronic diseases. Macrophage activation has been found to play an important role in the inflammatory process (Beutler, 2000) and to generate potent inflammatory mediators, such as tumor necrosis factor (TNF)-α, interleukin (IL)-6, cyclooxygenase (COX)-2, and inducible nitric oxide synthase (iNOS). The release of these mediators may be of major importance in the development of a variety of chronic inflammatory diseases, including allergic reactions, Crohn’s disease, and rheumatoid arthritis (Lind, 2003). Therefore, the suppression of inflammatory mediator levels is considered to be a potent treatment strategy for inflammation-related diseases.
Nuclear factor-kappa B (NF-κB) is a transcription factor and performs a crucial function in the expression of many genes involved in inflammatory responses, for example, TNF-α, IL-6, and COX-2 (Gadaleta et al., 2011). In response to inflammatory stimuli, the IκB is phosphorylated, ubiquinated, and degraded, allowing for NF-κB to translocate into the nucleus, where it can bind to the specific DNA sequences located in the promoter regions of target genes and modulate gene transcription. Recently, many studies have reported the role of NF-κB in inflammatory disorders (Gilmore and Garbati, 2011). It has been reported that increased activation of NF-κB has been found in macrophages and epithelial cells of patients with chronic inflammatory diseases (Wong et al., 2001). Therefore, NF-κB is currently regarded as an ideal target for molecular therapies to treat inflammation.
Caspase-1 is a member of a family of caspases with large prodomains, and its activation is involved in apoptosis and inflammation (Lee et al., 2001). Activation of caspase-1 induces the inflammatory response by producing pro- inflammatory cytokines and recruiting neutrophils (Wang et al., 2005). It has been reported that caspase-1 deficiency reduced the inflammation (Kuida et al., 1995).
Zanthoxylum piperitum D.C. (ZP) peels has been widely used as a natural food condiment as well as a traditional medicine. Especially, ZP has been traditionally used for prevention of stomachache, toothache, and anthelmintic problems (Choi et al., 2008; Park et al., 2008). It was reported that ZP contains various constituents including coumarins, lignans, flavonoids, quinolines, benzenoids, and triterpenoids (Ahsan et al., 2000; Tantapakul et al., 2012). These constituents have anti-oxidative and anti-platelet aggregation activities (Yamazaki et al., 2007). Nevertheless, the anti-inflammatory mechanisms employed by ZP have yet to be completely understood. In an effort to elucidate the mechanism responsible for ZP’s anti-inflammatory activity, we investigated the effects of ZP on the expression of inflammatory mediators and activation of NF-κB and caspase-1 in lipopolysaccharide (LPS)-induced RAW264.7 cells.
Materials and Methods
Reagents
We purchased 3-(4,5-dimethylthiazol-2-yl)-diphenyl-tetrazoliumbromide (MTT), bicinchoninic acid (BCA), LPS, dimethyl sulfoxide (DMSO), avidin peroxidase (AP) and other reagents from Sigma-Aldrich (St. Louis, MO, USA). Cell culture reagents and fetal bovine serum (FBS) were purchased from Gibco BRL (Grand Island, NY, USA). Anti- mouse IL-6/ TNF-α, biotinylated anti-mouse IL-6/ TNF-α and recombinant mouse IL-6/ TNF-αwere purchased from BD Pharmingen (San Diego, CA, USA).The specific Ab against iNOS, COX-2, NF-κB, and β-actin were purchased from Santa Cruz Biotechnology (Santa Cruz, CA, USA ).
Preparation of ZP
We purchased ZP from an oriental drug store, Daehak Oriental Drugstore (Iksan, Korea); it was identified by Professor Seung-Heon Hong of the Department of Oriental Pharmacy, Wonkwang University. An extract was prepared by decocting (Sunileyela, CCA-112, China) the dried prescription with boiling distilled water (100 g/L). The extraction was decocted for approximately 3 h, filtered (Whatman, GD/X, USA), lyophilized (Sunileyela, EYELA- FDU-1200, Japan), and kept at 4℃ (yield: 7.31%). The samples were dissolved in distilled water and then filtered through a 0.22 ㎛ syringe filter (Whatman, GD/X, USA).
Cell culture
Cells were cultured in Dulbecco’s Modified Eagle’s Medium (DMEM) containing 100 IU/mL penicillin, 100 ㎍/mL streptomycin, and 10% FBS at 37℃ in a 5% CO2 atmosphere at 95% humidity (N-BIOTEK, NB-203XL, KOREA).
MTT assay
To investigate the cell viability by ZP, we did an MTT colorimetric assay. Briefly, cells were incubated with ZP (0.01, 0.1, and 1 ㎎/mL) for 24 h, with 50 μL of MTT solution subsequently added, and were incubated for 4 h. Next, the crystallized MTT (formazan) was dissolved in 1 mL of DMSO, and the absorbance of the plate was read at 540 ㎚.
Cytokine assay
The TNF-α and IL-6 levels were evaluated by modification of an enzyme-linked immunosorbent assay (ELISA) as previously described (Kim et al., 2010). Briefly, 96-well plates were coated with anti-mouse TNF-α and IL-6 monoclonal Abs and incubated overnight at 4℃. After additional washes, a sample or standard solution of TNF-α and IL-6 was added and incubated for 2 h. Plates were exposed to biotinylated anti- mouse TNF-α and IL-6 Abs was added and incubated for 2 h. After washing plates, we sequentially added AP and 2,2′-azino-bis (3-ethylbenzthiazoline-6-sulfonic acid) substrate containing H2O2. Finally we evaluated, the optical density of plate at 405 ㎚ using a Micro reader (Molecular Devices Corporation, VersaMax, USA).
PGE2 assay
The PGE2 concentration by LPS-stimulated RAW264.7 macrophages was measured by PGE2 assay kit according to the manufacturer’s directions (Stressgen Biotechnologies, USA). Briefly, cell lysates and standard were put into different wells on the coated micro plate provided in the kit. Mouse anti-PGE2 antibody and peroxidase-conjugated PGE2 were added to each well, and the plate was incubated at room temperature and shaken for 2 h. The wells washed, and substrate solution was added and incubated at room temperature for 30 min. The reaction was stopped through the addition of stop solution. The absorbance was measured using Micro reader (Molecular Devices Corporation, VersaMax, USA) at 450 ㎚.
Measurement of nitrite (NO) concentration
Cells (3 × 105 cells/well) were pretreated with ZP for 1 h, and then treated with rIFN-γ (10 U/mL) and LPS (1 ㎍/mL) for 24h. The NO content secreted by cells was measured using Griess reagent. The cell-culture supernatant in 96-well plates was mixed with an equal volume of Griess reagent at room temperature for 15 min. The color developed was measured at 540 ㎚ using a Micro reader (Molecular Devices Corporation, VersaMax, USA). The concentration of NO was calculated from a standard curve generated using sodium nitrite.
Western blot analysis
For analysis of the level of indicated proteins, stimulated cells were rinsed twice with ice-cold PBS and then lysed in ice-cold lysis buffer (PBS containing 0.1% SDS, 1% triton, and 1% deoxycholate). Nuclear extracts were isolated by Nuclear Extraction Reagents (Pierce Thermo Scientific, Rockford, USA). Cell lysates were centrifuged at 15,000 × g for 5 min at 4℃; the supernatant was then mixed with an equal volume of 2 × SDS sample buffer, boiled for 5 min, and then separated through a 10% denaturing protein gel. After electrophoresis, the protein was transferred to nylon membranes by electrophoretic transfer. The membranes were blocked in 5% skim milk for 2 h, rinsed and incubated overnight at 4℃ with primary antibodies. After three washes in PBST/0.1% Tween 20, the membranes were incubated for 1 h with HRP-conjugated secondary antibodies. After three washes in PBST/0.1% Tween 20, the antibody-specific proteins were visualized using an enhanced chemiluminesence detection system purchased from Pierce Thermo Scientific (Rockford, IL, USA).
Luciferase reporter gene assay
RAW264.7 cells were transiently transfected with NF-κB-luc DNA and refreshed with completed DMEM. The transfected cells were seeded in 6-well plates overnight and pretreated with ZP before LPS stimulation for another 2 h. Luciferase activity was detected using a Dual-Glo luciferase assay system kit (Promega, Madison, Wisconsin, USA) following the manufacturer’s instructions.
Caspase-1 activity assay
The enzymatic activity of caspase-1 was assayed using a caspase colorimetric assay kit according to the manufacturer’s protocol (R & D systems, Minneapolis, USA). The lysed cells were centrifuged at 14,000 rpm for 5 min. The protein supernatant was incubated with 50 μL reaction buffer and 5 μL caspase substrate at 37℃ for 2 h. The absorbance was measured using a Micro reader (Molecular Devices Corporation, VersaMax, USA) at a wavelength of 405 nm. Equal amounts of the total protein from each lysate were quantified using a BCA quantification kit.
Statistical analysis
The results are presented as the mean ± S.E.M. of at least three experiments. The results were examined using an independent t- tests and ANOVA with a Tukey post hoc test. A p < 0.05 was considered significant.
Results
ZP inhibits production of TNF-α and IL-6 in LPS-induced RAW264.7 cells
Generally, the inhibition of TNF-α and IL-6 production is considered to be a potent treatment strategy for inflammatory diseases. To investigate the anti-inflammatory activity of ZP, we evaluated the effects of ZP on TNF-α and IL-6 production from LPS-stimulated RAW264.7 cells. As shown in Fig. 1, the production of TNF-α and IL-6 in response to LPS was inhibited via pre-treatment with ZP (0.01, 0.5, and 1 ㎎/mL). The maximal inhibition rates of TNF-α and IL-6 production by ZP (1 ㎎/mL) were approximately 46.1% (p < 0.05) and 40.2% (p < 0.05), respectively. No cell cytotoxicity by ZP was observed (data not shown).
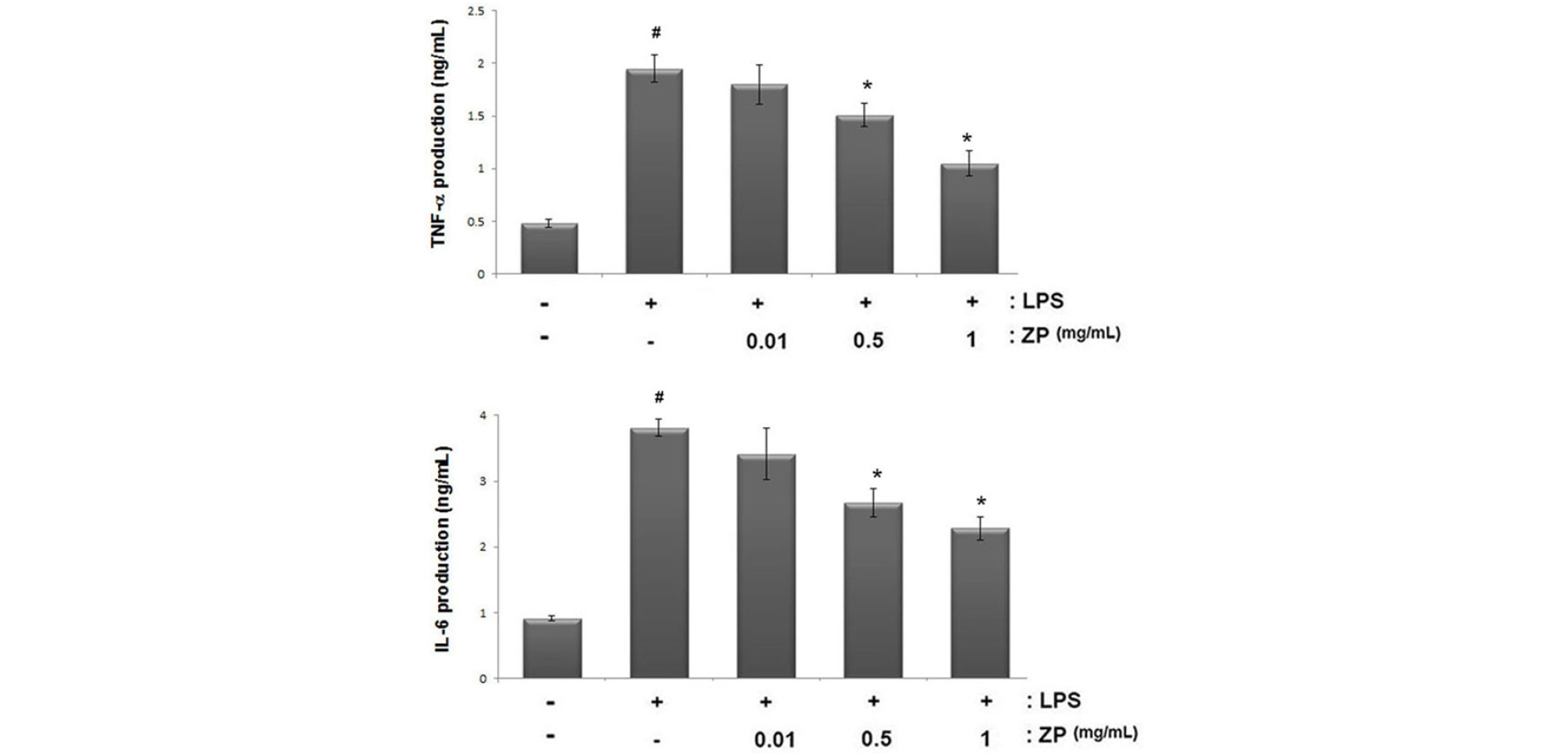
Fig. 1.
Effect of ZP on TNF-α and IL-6 production in LPS-stimulated RAW264.7 cells. Cells (3 × 105 cells/mL) were pretreated for 1 h with ZP (0.1, 0.5 and 1 ㎎/mL) and then stimulated for 24 h with LPS (1 ㎍/mL). The levels of TNF-α and IL-6 in the supernatant were measured via ELISA. All data are expressed as the means ± SEM of three independent experiments. (#P < 0.05 vs. control, *P < 0.05 vs. LPS alone)
ZP inhibits production of PGE2 and expression of COX-2 in LPS-induced RAW264.7 cells
During inflammation, an increase of COX-2 levels is associated with the physiological processes of inflammation. In this study, we used Western blot analysis to assay the effects of ZP on LPS-induced COX-2 expression. The cells were pretreated for 1 h with ZP (0.01, 0.5, and 1 ㎎/mL) and then treated for 24 h with LPS. Our results showed that LPS increased the levels of COX-2 expression more than in un-stimulated cells. However, ZP inhibited the increased COX-2 levels in LPS-stimulated RAW264.7 cells. The relative level of COX-2 is represented in Fig. 2B.
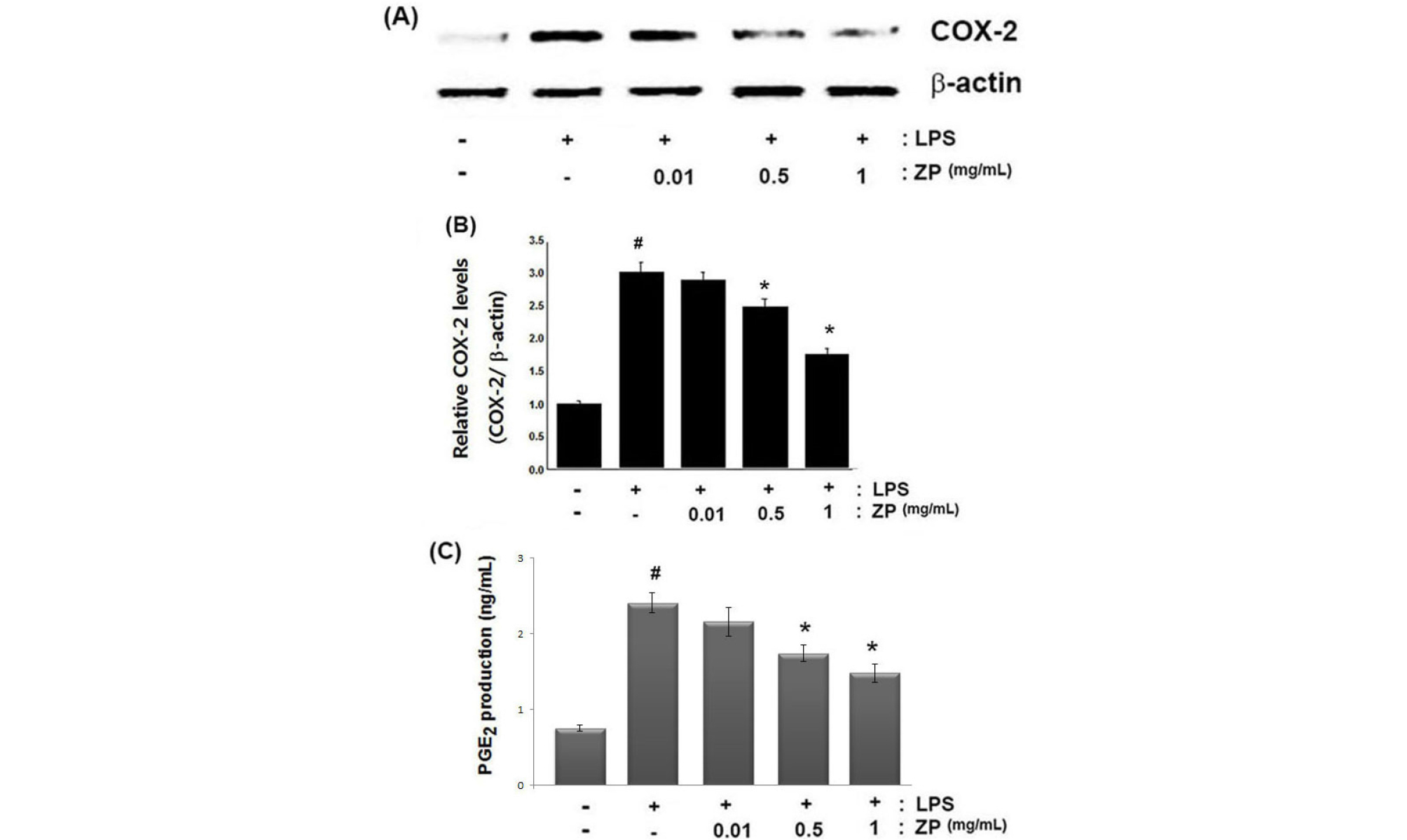
Fig. 2.
Effects of ZP on COX-2 expression and PGE2 production in LPS-stimulated RAW264.7 cells. (A) Cells were pretreated for 1 h with ZP (0.1, 0.5 and 1 ㎎/mL) and then stimulated for 24 h with LPS (1 ㎍/mL). The protein extracts were assayed via Western blot analysis for COX-2. (B) The relative level of COX-2 was measured using an image analyzer. (C). Cells were pretreated for 1 h with ZP (0.1, 0.5 and 1 ㎎/mL) and then stimulated for 24 h with LPS (1 ㎍/mL). The amount of PGE2 production was measured with immunoassay kits. All data represent the means ± SEM of three independent experiments. (#P < 0.05 vs. control, *P < 0.05 vs. LPS alone)
COX-2 catalyzes the biosynthesis of PGE2,which contributes to the pain and swelling in inflammatory disease. Thus, we examined how ZP inhibits PGE2 production. As shown in Fig. 2C, PGE2 production was increased in response to LPS treatment; however, this increase was inhibited significantly by ZP pretreatment in a dose-dependent manner. The maximal inhibition rate of PGE2 production by ZP (1 ㎎/mL) was approximately 38.6% (p < 0.05).
ZP inhibits production of NO and expression of iNOS in LPS-induced RAW264.7 cells
Because overexpression of NO is involved in the inflammatory process, we investigated the effects of ZP on LPS-induced NO production. Cells were pretreated for 1 h with ZP (0.01, 0.5, and 1 ㎎/mL) and then stimulated with rIFN-γ (10 U/mL) and LPS (1 ㎍/mL). The resultant NO production was then assessed using Griess reagent. The results revealed that ZP induced a reduction in NO production in a dose-dependent manner (Fig. 3A), with the maximal inhibition rate of NO production by ZP (1 ㎎/mL) being measured as 40.9% (p < 0.05). Next, we did Western blot analysis to measure the effect of ZP on LPS-induced iNOS expression in murine macrophages. As shown in Fig. 3B, LPS treatment induced a significant increase in iNOS expression; however, the level of iNOS decreased in response to treatment by ZP. The relative level of iNOS is represented in Fig. 3C.
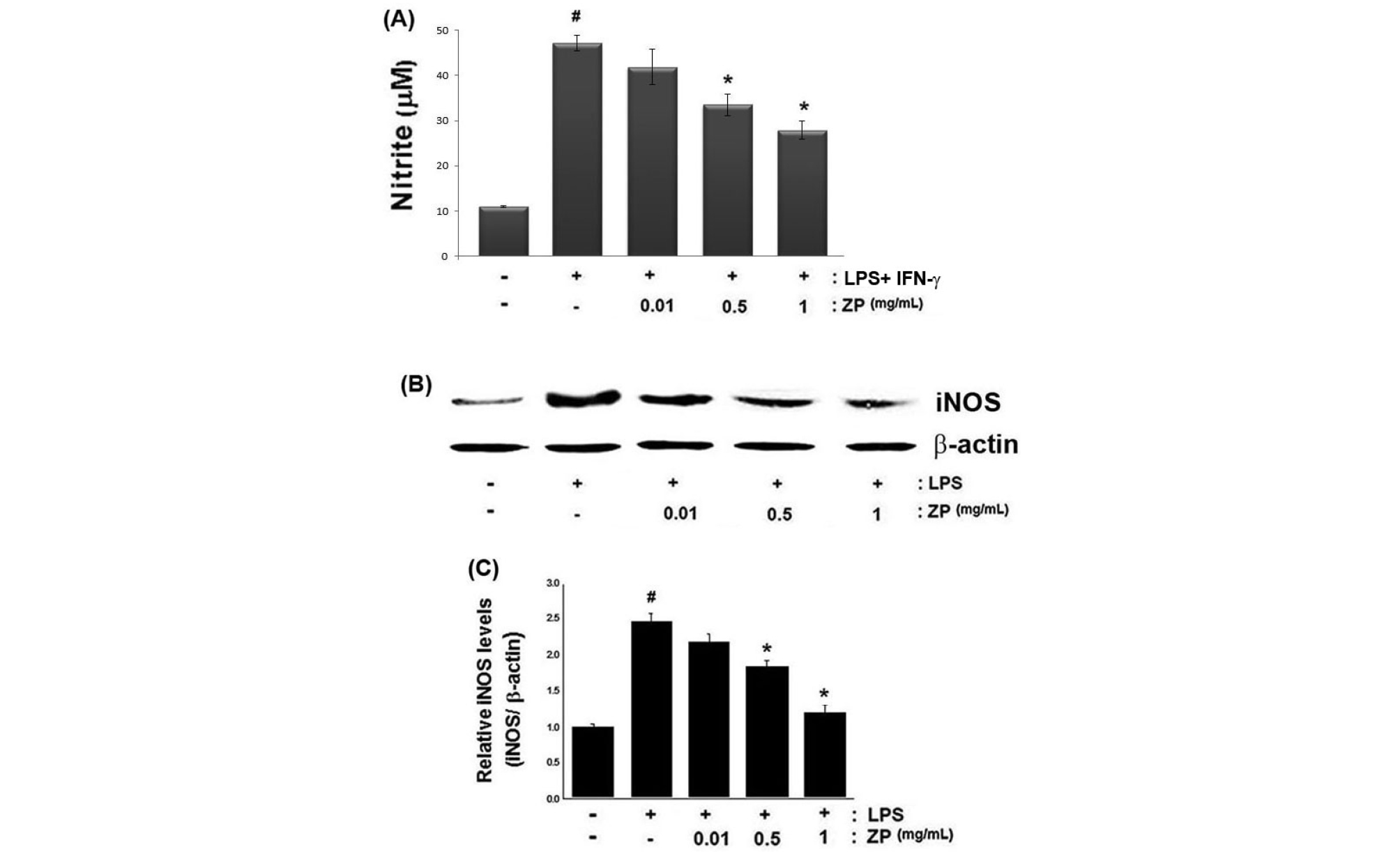
Fig. 3.
Effects of ZP on NO production and iNOS expression in LPS-stimulated RAW264.7 cells. Cells were pretreated for 1 h with ZP (0.1, 0.5 and 1 ㎎/mL) followed by 6 h of treatment with rIFN-γ (10 U/mL), and 48 h of LPS stimulation (1 ㎍/mL). (A) NO production in the medium was measured via the Griess reagent. The amount of NO production was quantitatively assessed using NaNO2 as a standard. (B) The protein extracts were assayed by Western blot analysis for iNOS. (C) The relative level of iNOS was measured using an image analyzer. All data are expressed as the means ± SEM of three independent experiments. (#P < 0.05 vs. control, *P < 0.05 vs. LPS alone)
ZP inhibits NF-κB activation in LPS-induced RAW264.7 cells
NF-κB is an important regulator of various genes involved in inflammatory responses. Because NF-κB activation requires nuclear translocation of NF-κB (RelA/p65), we evaluated the effects of ZP on the nuclear pool of RelA/p65 protein levels via Western blot analysis. In this study, we observed that the levels of Rel/p65 were increased in the nucleus, but ZP reduced these increased levels of Rel/p65 in LPS-stimulated cells, (Fig. 4A). The relative level of Rel/p65 is represented in Fig. 4B. Additionally, the promoter activity of NF-κB was evaluated using a luciferase reporter assay. As illustrated in Fig. 4C, ZP significantly attenuated the NF-κB-driven luciferase activity in LPS-stimulated RAW264.7 cells.
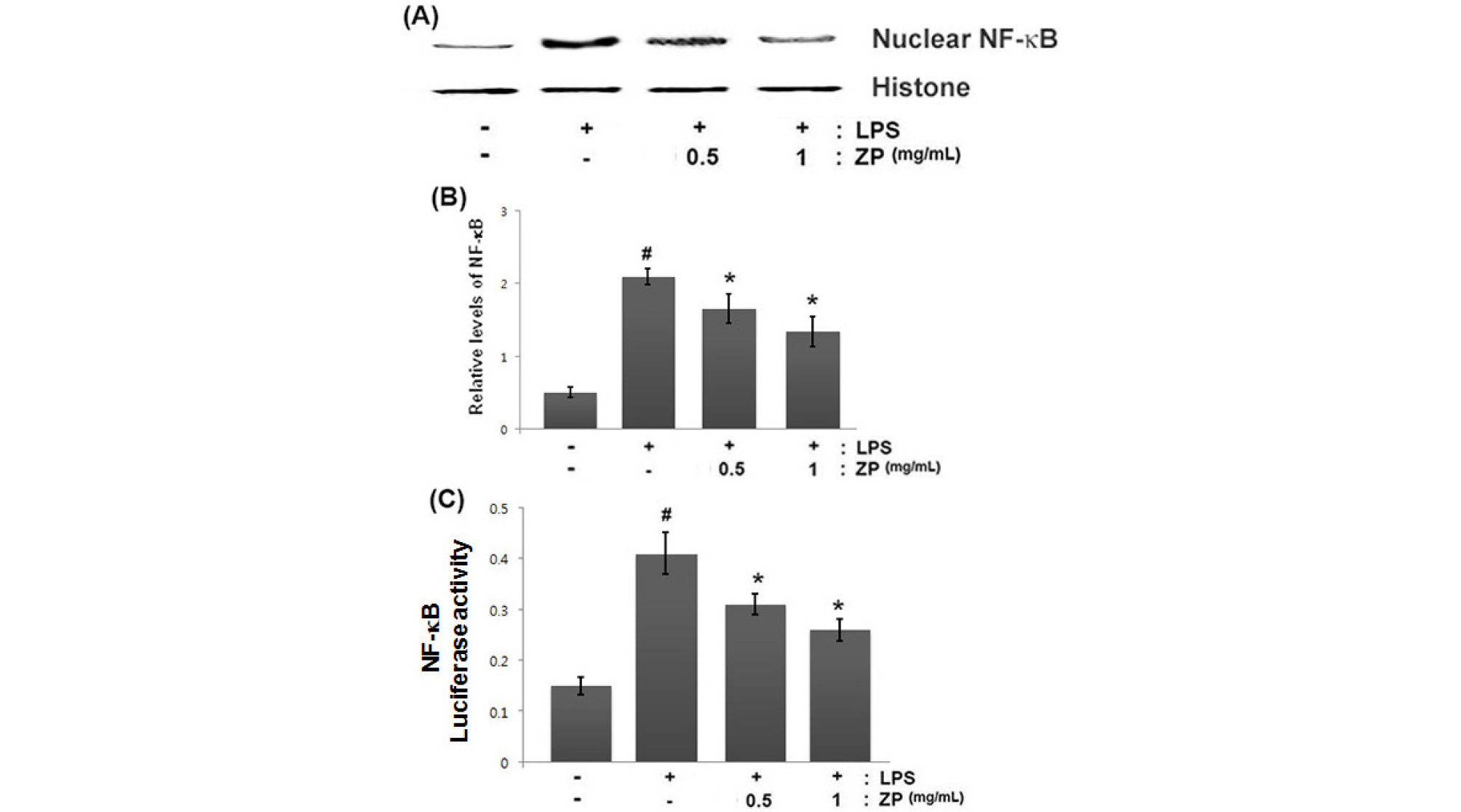
Fig. 4.
Effect of ZP on NF-κB activation in LPS-stimulated RAW264.7 cells. (A) Cells were pretreated for 1 h with ZP (0.1, 0.5 and 1 ㎎/mL) and then treated for 2 h with LPS (1 ㎍/mL). Nuclear extracts were prepared as described in the Materials and Methods section and evaluated for RelA/p65 via Western blot analysis. (B) The relative expression level of RelA/p65 was measured using an image analyzer. (C) Luciferase activity was determined using a luciferase assay system kit following the manufacturer’s instructions. All data are expressed as the means ± SEM of three independent experiments. (#P < 0.05 vs. control, *P < 0.05 vs. LPS alone)
ZP inhibits caspase-1 activation in LPS-induced RAW264.7 cells
Activation of caspase-1 is associated with inflammation and production of inflammatory mediators. To identify the anti-inflammatory mechanism of ZP, we investigated whether ZP could suppress the activation of caspase-1 in LPS-stimulated cells. The cells were pretreated for 1 h with ZP (0.01, 0.5, and 1 ㎎/mL) and then treated for an additional 12 h with LPS. We evaluated the effects of ZP on caspase-1 activation using a caspase-1 assay kit. As shown in Fig. 5, LPS treatment induced the caspase-1 activation. However, the increased caspase-1 activity was down-regulated by treatment of ZP in a dose-dependent manner.
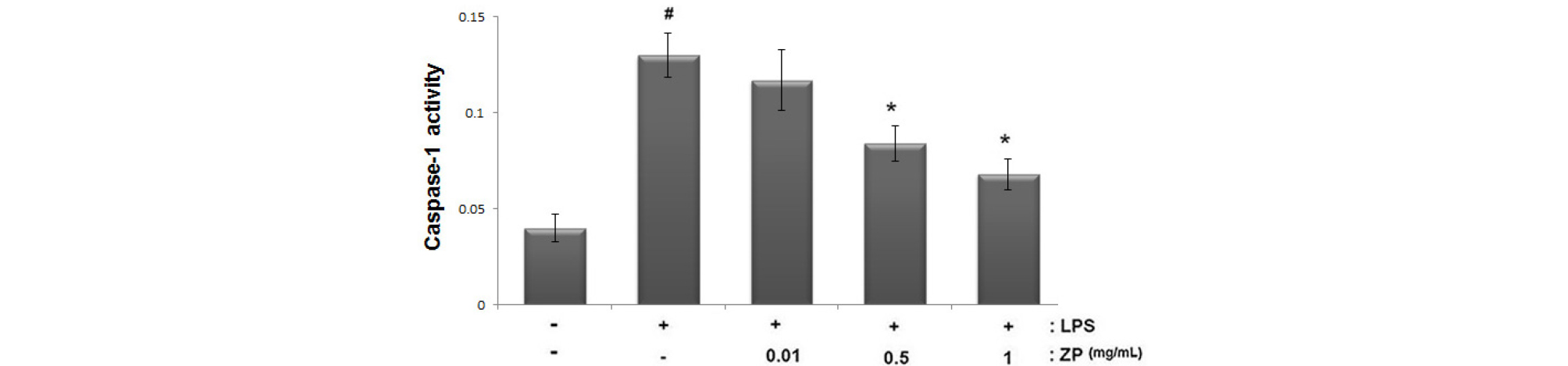
Fig. 5.
Effect of ZP on caspase-1 activation in LPS-stimulated RAW264.7 cells. The cells were pretreated with ZP (0.1, 0.5 and 1 ㎎/mL) for 1 h prior to LPS stimulation for 12 h. The enzymatic activity of caspase-1 was tested by a caspase colorimetric assay. All data are expressed as the means ± SEM of three independent experiments. (#P < 0.05 vs. control, *P < 0.05 vs. LPS alone)
Discussion
ZP is a natural spice and Korean medication that has traditionally been used to treat a variety of diseases. However, the exact anti-inflammatory mechanism of ZP has yet to be thoroughly elucidated. The findings of this study show that ZP reduced the production of TNF-α, IL-6, NO, and PGE2 and the expression of COX-2 and iNOS. In addition, this anti-inflammatory effect of ZP is by regulating NF-κB and caspase-1 activation in LPS-induced RAW264.7 cells. A model of the anti-inflammatory mechanism of ZP has been provided in Fig. 6. This result suggests an important molecular mechanism by which pZP ameliorates inflammatory reaction.
The inflammatory process is an important immunological response induced by many factors, including pathogens, injury, organic compounds, and endotoxins (Fedenko et al., 2011). The resulting inflammatory responses involve macrophages activation. Macrophages actively participate in inflammatory responses by releasing inflammatory mediators and cytokines (Hawiger, 2001). TNF-α, and IL-6 are potent inflammatory cytokines that are rapidly elevated in response to inflammatory triggers (Bunikowski et al., 2001). PGE2 is an inflammatory mediator produced at inflammatory sites by COX-2 that contributes to the pain and swelling associated with inflammation (Tegeder et al., 2001). Although NO has been shown to perform a crucial role in host defenses against a variety of pathogens, overproduction of NO has been proven to be harmful and may result in septic shock, rheumatoid arthritis, and autoimmune diseases (Leiro et al., 2004). Therefore, therapeutic agents that inhibit iNOS may be useful in relieving such inflammatory conditions. These results suggested that suppression of macrophages cell-mediated inflammatory mediators has been identified as a useful effect for the treatment of inflammatory diseases. Another study reported that stimulation of RAW264.7 cells with LPS has been used to evaluate the regulatory effects of natural herbs (Woo et al., 2018). Therefore, we attempted to investigate whether ZP’s anti-inflammatory effect takes place by suppressing inflammatory mediators such as COX-2, PGs and NO in mouse macrophages. We focused on how ZP regulates the inflammatory mediators in LPS-simulated RAW264.7 cells. In this study, we showed that ZP significantly inhibited the secretion of inflammatory cytokines (TNF-α, and IL-6) and mediators (PGE2, COX-2, NO, and iNOS) induced by LPS. From this, we propose that the anti-inflammatory activity of ZP may be associated with the regulation of inflammatory-related gene expression.
Accumulating evidence suggested that the NF-κB performs a crucial function by regulating the expression of various genes involved in inflammatory responses (Shin et al., 2019). In the nucleus, NF-κB activates gene transcription; thus, NF-κB is important in the regulation of inflammatory responses, by controlling the transcription of inflammatory genes (e.g., TNF-α, IL-6, and COX-2) (Kanigur Sultuybek et al., 2019). For this reason, extensive efforts have been made to develop new treatments that target NF-κB. It was reported that increased NF-κB activity associated with the production of high levels of inflammatory genes was involved in inflammation. Based on these results, we identified suppression of NF-κB activation as an anti-inflammatory strategy. Therefore, we examined whether the anti-inflammatory mechanism of ZP occurs by regulating NF-κB activation. In this study, we observed that the ZP significantly attenuated the NF-κB translocation into the nucleus and NF-κB-driven luciferase activity in LPS-stimulated RAW264.7 cells. Therefore, we hypothesized that ZP might exert its anti-inflammatory mechanism via NF-κB activation.
Caspase-1 is a member of the caspase family and plays an important role in apoptosis and inflammation (Bouchier-Hayes and Martin 2004). Activation of caspase-1 is associated with an increase of inflammatory mediators such as cytokines.It was reportedthat caspase-1 deficiency in mice reduced the cytokine production (Kuida et al., 1995). Additionally, it was revealed that activation of caspasse-1 induced NF-κB and MAPK-signaling pathways (Siegmund et al., 2001; Lamkanfi et al., 2004). Therefore, we postulated that the anti-inflammatory mechanism of ZP is mediated, at least in part, by suppressing caspase-1 activation. In this study, we noted that ZP suppressed the LPS-induced activation of caspase-1. This finding demonstrated that ZP might inhibit inflammation by blocking caspase-1 activation in LPS-stimulated RAW264.7 cells.
In conclusion, our results demonstrated that the anti- inflammatory activities of ZP could be attributed, at least in part, to the inhibition of inflammatory mediators. This anti- inflammatory mechanism of ZP works by down-regulating LPS-induced NF-κB activation and caspase-1 activation. These results provide experimental evidence that ZP might be a potential candidate for treating inflammatory diseases.