Introduction
Materials and Methods
Bacterial strains and culture conditions
Plant materials
Hodge test
Antimicrobial susceptibility assay
β-Lactamase activity assay
Statistical analysis
Results
Antibiotic susceptibilities of SAWT, SACIP, SAOXA, and SACLI in the presence of medicinal plant extracts
Inhibitory effect of medicinal plant extracts on the production of β-lactamases by SAWT, SACIP, SAOXA, and SACLI
Discussion
Abbreviations
Introduction
Over the last few decades, the misuse and overuse of antibiotics has contributed to the development and rapid spread of multidrug-resistant (MDR) bacteria, leading to global threat due to the increased therapeutic failures (Lamers et al., 2013). MDR bacteria exposed to antibiotics have developed their own survival strategies underlying several mechanisms such as enzyme production and efflux pump activity (Rumboet al., 2013). The development of resistance to β-lactam antibiotics in Staphylococcus aureus is mainly attributed to the production of β-lactamases (Sibanda and Okoh, 2007). S. aureus produces β-lactamases that hydrolyze β-lactam antibiotics, responsible for the variants of methicillin-resistant S. aureus (MRSA) (Fuda et al., 2005). In addition, the mutational alterations in and overproduction of penicillin-binding proteins (PBPs) result in the increased resistance to β-lactam antibiotics (Sun et al., 2014). The discovery of new classes of antibiotic has become a global priority. However, the development of novel antibiotics has been faced with difficulty because it lags far behind the rapid evolution of antibiotic resistance in bacteria. Therefore, novel strategy for tackling antibiotic resistance problem is urgently needed to protect global public health.
Recently, potentiators have gained growing attention towards potential adjuvants used in combination with antibiotics (Zabawa et al., 2016). The potentiators act as inhibitors of β-lactamases, known as β-lactamase inhibitors (BLIs) such as clavulanate, sulbactam, and tazobactam (Bush and Bradford, 2016). The combined treatments of β-lactams and BLIs can extend the spectrum of antibiotics against not only Gram-positive bacteria but also Gram-negative bacteria (Sood, 2013). BLIs are commercially used for enhancing broad-spectrum activity, including ampicillin/sulbactam, cefoperazone/sulbactam, piperacillin/tazobactam, ticarcillin/clavulanate, cefepime/clavulanate, and carbapenem/clavulanate (Akova, 2008; Cheng et al., 2019). In addition to the enhanced antibiotic activity, BLIs can extend the use of conventional antibiotics by re-considering sub-potent and outdated antibiotics (Zabawa, et al., 2016). Medicinal plants, which show antimicrobial properties, are well recognized for antibiotic development with less or no side effects (Lee et al., 2019; Rubens et al., 2015). The screening of plant extracts have gained more attention for the discovery of novel drugs in the treatment of life-threatening bacterial infections. Medicinal plants are desirable to consider possible sources of BLIs due to the increasing demand for naturally occurring antibiotics (Aparna et al., 2014). Therefore, the objective of this study was to assess the possibility of using medicinal plants as BLIs in combination with antibiotics that can fight antibiotic-resistant S. aureus.
Materials and Methods
Bacterial strains and culture conditions
Strains of Staphylococcus aureus ATCC 15564 (SAWT) and clinically-isolated S. aureus CCARM 3008 (SACLI) were obtained from American Type Culture Collection (ATCC, Manassas, VA, USA) and Culture Collection of Antibiotic Resistant Microbes (CCARM, Seoul, Korea), respectively. SAWT was serially exposed to stepwise increasing concentrations of ciprofloxacin and oxacillin to induce antibiotic-resistant strains (Michéa-Hamzehpour et al., 1994), which were assigned as ciprofloxacin-induced S. aureus ATCC 15564 (SACIP) and oxacillin-induced S. aureus ATCC 15564 (SAOXA), respectively. All strains were cultured in typical soy broth (TSB; BD, Becton, Dickinson and Co., Sparks, MD) at 37℃ for 20 h. The cultured cells were centrifuged with phosphate-buffered saline (PBS, pH 7.2) at 6,000 × g for 10 min at 4℃ to remove cell debris and then suspended in PBS to a concentration of 108 cfu/mL. No noticeable changes in the antibiotic resistance profiles of SACIP and SAOXA were observed through 10 serial passages in antibiotic-free TSB.
Plant materials
The extracts of medicinal plants, including Cleyera japonica (CJ), Carpinus laxiflora (CL), Euphorbia helioscopia (EH), Euscaphis japonica (EJ), Oenothera erythrosepala (OE), and Rosa multiflora (RM) were kindly provided by Dr. Kil-Nam Kim from the Korea Basic Science Institute (KBSI, Chuncheon, Korea). All medicinal plants were extracted with water at 60℃ for 24 h. The extracts were lyophilized using a freeze-dryer and stored at -20℃ prior to use. The extracts were dissolved in water to obtain 30 ㎎/mL of stock solutions.
Hodge test
Modified Hodge test was used to evaluate the β-lactamase-producing ability of SAWT, SACIP, SAOXA, and SACLI in the presence of cefoxitin, ceftriaxone, imipenem, meropenem, ampicillin, and piperacillin (Amjad et al., 2011; Anderson et al., 2007). In brief, Escherichia coli as a control strain was evenly spread on the surface of Mueller-Hinton (MH) agar. The spread-plated MH agar was cut straightly from the center to the edge using a blade smeared with SAWT, SACIP, SAOXA, and SACLI. Antibiotic discs, including cefoxitin (30 ㎍), ceftriaxone (30 ㎍), imipenem (10 ㎍), meropenem (10 ㎍), ampicillin (10 ㎍), and piperacillin (100 ㎍), were placed on the surface of MH agar and then incubated at 37℃ for 20 h. Distortion in the clear zone of inhibition was observed to determine the production of β-lactamases.
Antimicrobial susceptibility assay
The susceptibilities of SAWT, SACIP, SAOXA, and SACLI to ampicillin in the absence and presence of medicinal plant extracts, including CJ, CL, EH, EJ, OE, and RM, were determined using a broth microdilution assay according to the Clinical Laboratory Standards Institute (CLSI) procedure (CLSI, 2015). The ampicillin (100 µL) was serially (1:2) diluted with TSB in 96-well microtiter plates. Approximately 105 cfu/mL of each strain were inoculated, and the predetermined quarter MIC of each extract (MICs = 0.4-15 ㎎/mL) was added in the 96-well microtiter plates. The prepared microtiter plates were incubated at 37℃ for 20 h. MICs were determined at the lowest concentration where no growths were visually observed.
β-Lactamase activity assay
The β-lactamase activity of SAWT, SACIP, SAOXA, and SACLI was evaluated by using a nitrocefin-hydrolyzing assay (Matsumoto et al., 2011). Each strain was cultured in the absence and the presence of a quarter MIC of medicinal plant extracts (CJ, CL, EH, EJ, OE, and RM) at 37℃ for 20 h. BLI-489 was used as a positive β-lactamase inhibitor. The cultures were centrifuged at 6000 × g for 10 min at 4℃. The cell-free supernatants were mixed with 10 μL of 1.5 mM nitrocefin and incubated at 37℃ for 30 min. The absorbance was measured every 5 min at 515 ㎚ using a microplate reader.
Statistical analysis
All experiments were conducted in three replicates. The collected data were analyzed by the Statistical Analysis System (SAS). The General Linear Model (GLM) and least significant difference (LSD) procedures were used to compare treatments at p < 0.05.
Results
Antibiotic susceptibilities of SAWT, SACIP, SAOXA, and SACLI in the presence of medicinal plant extracts
The β-lactamase-producing strains were screened by the modified Hodge assay (Fig. 1). Antibiotics are hydrolyzed by β-lactamases produced by the test strains (SAWT, SACIP, SAOXA, and SACLI), resulting in the growth of sensitive control strain (E. coli). No noticeable phenotypic change in the clear inhibition zones around cefoxitin, ceftriaxone, imipenem, meropenem, and piperacillin discs was observed at all test strains. In contrast, the distortion in the clear zone of inhibition around ampicillin disc was observed at SAWT, SACIP, and SAOXA, except SACLI. The ampicillin susceptibilities of SAWT, SACIP, SAOXA, and SACLI were evaluated in the absence and presence of medicinal plant extracts, including Cleyera japonica (CJ), Carpinus laxiflora (CL), Euscaphis japonica (EJ), Euphorbia helioscopia (EH), Oenothera erythrosepala (OE), and Rosa multiflora (RM) (Table 1). SAOXA and SACLI (MIC=32 ㎍/mL) were highly resistance to ampicillin in the absence of medicinal plant extracts, followed by SACIP (MIC=16 ㎍/mL) and SAWT (MIC=4 ㎍/mL). SAWT, SACIP, SAOXA, and SACLI were susceptible to all medicinal plant extracts with the exception of EH against SACLI, showing no change in the MIC (32 ㎍/mL). The MICs of ampicillin against SAWT, SACIP, SAOXA, and SACLI were decreased from 2 to 8 folds, 2 to 4 folds, 8 to16 folds, and 1 to 2 folds, respectively, in the presence of CJ, CL, EH, EJ, OE, and RM.
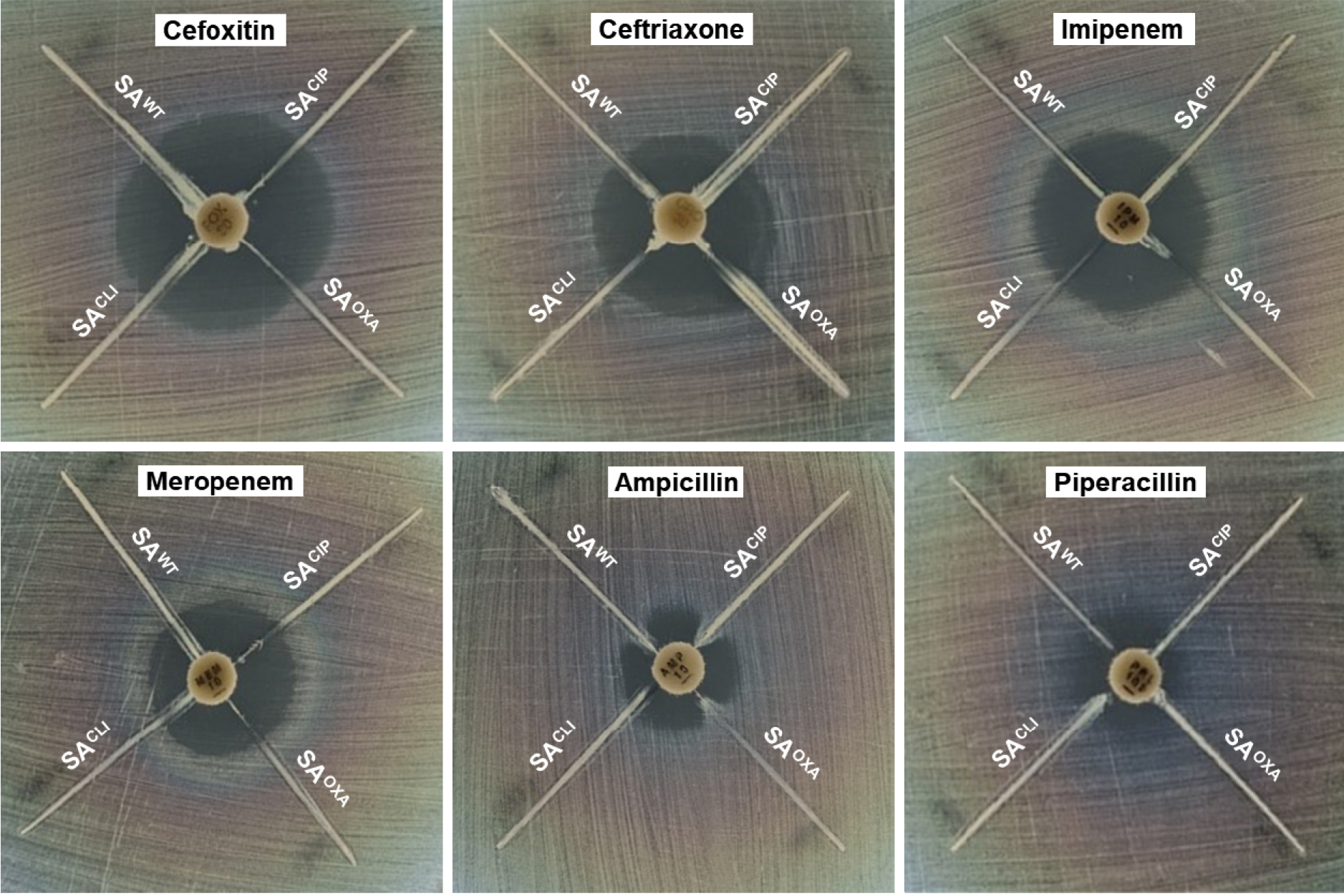
Fig. 1
Modified Hodge test for β-lactamase-producing Staphylococcus aureus ATCC 15564 (SAWT), ciprofloxacin-induced S. aureus ATCC 15564 (SACIP), oxacillin-induced S. aureus ATCC 15564 (SAOXA), and clinically-isolated S. aureus CCARM 3008 (SACLI) exposed to different antibiotics, including cefoxitin, ceftriaxone, imipenem, meropenem, ampicillin, and piperacillin.
Table 1.
MICs of ampicillin against Staphylococcus aureus ATCC 15564 (SAWT), ciprofloxacin-induced S. aureus ATCC 15564 (SACIP), oxacillin-induced S. aureus ATCC 15564 (SAOXA), and clinically-isolated S. aureus CCARM 3008 (SACLI) treated without and with medicinal plant extractsz
Strain | Ampicillin | Ampicillin with plant extractz | |||||
CJ | CL | EH | EJ | OE | RM | ||
SAWT | 4 | 1 | 0.5 | 2 | 2 | 0.5 | 1 |
SACIP | 16 | 8 | 8 | 8 | 8 | 8 | 4 |
SAOXA | 32 | 4 | 2 | 2 | 4 | 4 | 2 |
SACLI | 32 | 16 | 16 | 32 | 16 | 16 | 16 |
Inhibitory effect of medicinal plant extracts on the production of β-lactamases by SAWT, SACIP, SAOXA, and SACLI
The extracellular β-lactamase activities of SAWT, SACIP, SAOXA, and SACLI were estimated in the presence of CJ, CL, EH, EJ, OE, and RM, compared to the control (Fig. 2). The highest β-lactamase activities were observed in SAWT, SACIP, and SAOXA in the absence of medicinal plant extracts, showing 39, 32, and 43 µmol/min/mL, respectively. SACLI exhibited the lowest β-lactamase activity (3 µmol/min/mL), which was not significantly changed in the presence of CJ, CL, EH, EJ, OE, and RM (p > 0.05). The β-lactamase inhibitor, BLI-489, used as a positive control effectively reduced the β-lactamase activities of SAWT, SACIP and SAOXA up to less than 7 µmol/min/mL (> 80% reduction).
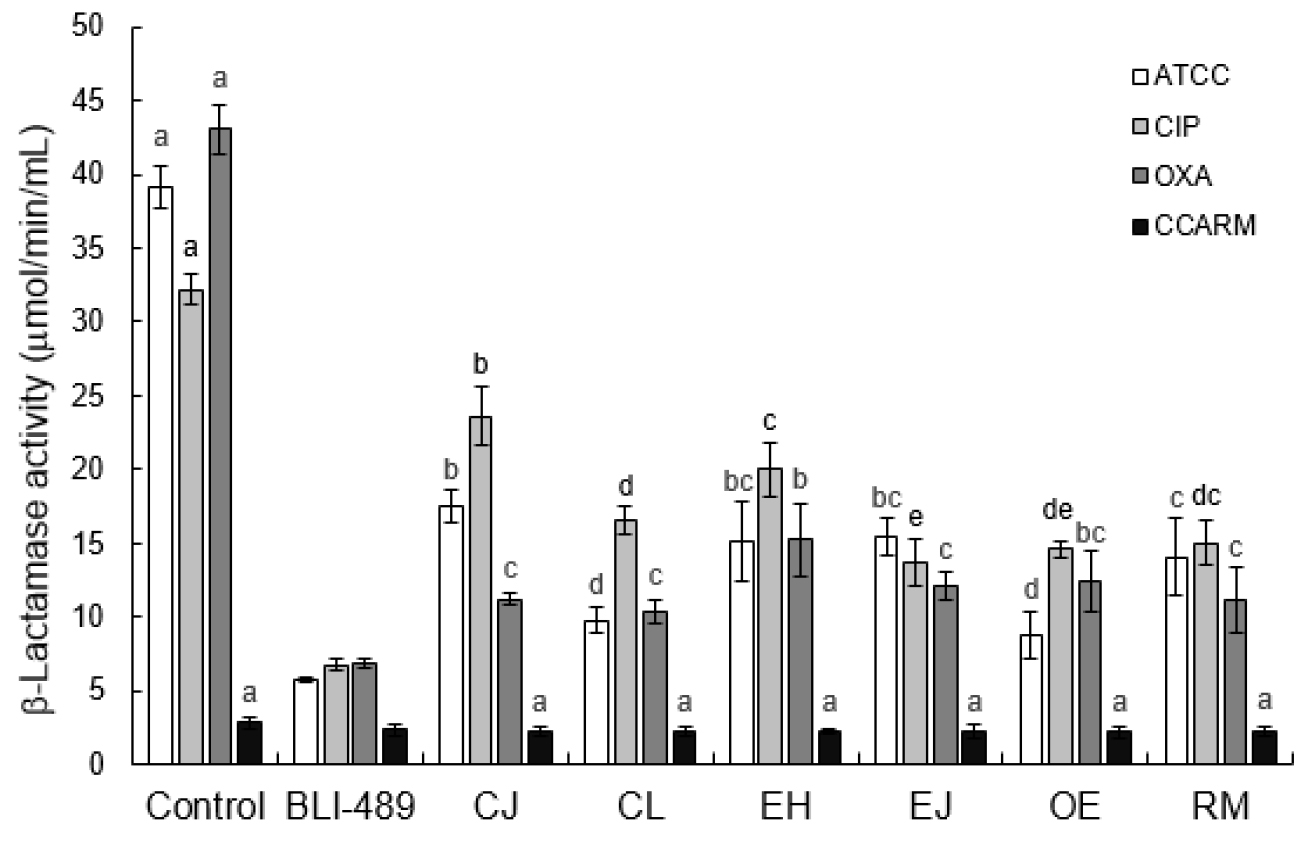
Fig. 2
Hydrolyzing activity of β-lactamases produced by Staphylococcus aureus ATCC 15564 (SAWT), ciprofloxacin-induced S. aureus ATCC 15564 (SACIP), oxacillin-induced S. aureus ATCC 15564 (SAOXA), and clinically-isolated S. aureus CCARM 3008 (SACLI) treated with BLI-489 and medicinal plant extracts (Cleyera japonica, CJ; Carpinus laxiflora, CL; Euphorbia helioscopia, EH; Euscaphis japonica, EJ; Oenothera erythrosepala, OE; Rosa multiflora, RM). Letters (a-c) on the bars are significantly different among treatments within each strain at p < 0.05.
Discussion
Since some bacteria can evolve antibiotic resistance mediated by the production of β-lactamases, the discovery of natural β-lactamase inhibitors can provide a clue for the control of antibiotic-resistant pathogens. Novel β-lactamase inhibitors can help to make the best reuse of unnoticed and outdated antibiotics.
The distortion in the clear zone of inhibition around ampicillin disc (Fig. 1) suggests that the reduced susceptibility of E. coli to ampicillin was mediated by ampicillin-hydrolyzing enzymes produced by SAWT, SACIP, and SAOXA, whereas SACLI did not produce β-lactamases. As a result, ampicillin was selected for further β-lactamase inhibition study. The specific types of β-lactamases, such as penicillinases and cephalosporinases, have their own specificity for β-lactam antibiotics based on the substrate specificity (Bauernfeind, 1986). The β-lactamases are divided into four classes, A (Bla-A, SHV, and TEM), B (IMP, SPM, and VIM), C (AmpC, Bla-B, and FOX), and D (OXA), and also classified into class I through VI (Barry and Barlow, 2005; Bauernfeind, 1986). The class A β-lactamases (class II, III, and V) are resistant to ESBLs, associated with the overexpression of AmpC and TEM (Féria et al., 2002). The class B β-lactamases are resistant to most β-lactam antibiotics (carbapenem) and β-lactamase inhibitors (clavulanic acid) (Hanson and Sanders, 1999; Payne et al., 1994). The class C β-lactamases (class I) include an inducible cephalosporinase (Hanson and Sanders, 1999; Manchanda and Singh, 2003). The class D β-lactamases (class IV) include OXA β-lactamase (Bauernfeind, 1986).
Previous studies reported that the medicinal plant extracts, CJ, CL, EH, EJ, OE, and RM, inhibited the growth of Helicobacter pylori, Listeria monocytogenes, S. aureus, S. epidermidis, Salmonella enterica, and Escherichia coli (Cendrowski et al., 2020; Elizaquível et al., 2013; Hou et al., 2003). The antimicrobial activity of CJ, CL, EH, EJ, OE, and RM was not considered in this study because the ampicillin treatments were combined with a quarter MIC of each extract that might not affect the viability of SAWT, SACIP, SAOXA, and SACLI. The decrease in the MICs of ampicillin combined with CJ, CL, EH, EJ, OE, and RM might be due to the inhibition of β-lactamase activity and/or PBP2a (Catteau et al., 2017). The medicinal plant extracts containing flavonoids, tannins, flavan-3-oleflavonoid, and epicatechin gallate can inhibit β-lactamase activity, leading to the inhibition of hydrolysis of β-lactam antibiotics (Eumkeb et al., 2012; Stapleton et al., 2004; Zhao et al., 2002). Therefore, the increased susceptibilities of SAWT, SACIP, and SAOXA to ampicillin in the presence of medicinal plant extracts imply that the medicinal plant extracts used in this study acted as β-lactamase inhibitor. However, the ampicillin-resistant SACLI (32 ㎍/mL) might be more associated with modified PBPs (PBP2a) that contributes to the resistance to β-lactam antibiotics, rather than the production of β-lactamases (Llarrull et al., 2009).
The β-lactamase inhibitor, BLI-489, effectively reduced the β-lactamase activities of SAWT, SACIP and SAOXA (Fig. 2). BLI-489 is a broad-spectrum β-lactamase inhibitor, which is effective against class A, C, and D β-lactamases when combined with piperacillin (Venkatesan et al., 2004). The common β-lactamase inhibitors, sulbactam and clavulanic acid, effectively inhibit the activity of extended-spectrum β-lactamases (ESBLs), but less effective against AmpC (Liu et al., 2016). The ability of CJ, CL, EH, EJ, OE, and RM to inhibit β-lactamases varied with strains used in this study (Fig. 2). The intrinsic β-lactamase activities of SAWT, SACIP, and SAOXA were decreased by 55-78%, 27-57%, and 65-76%, respectively, in the presence of medicinal plant extracts (Fig. 2). The highest reductions in β-lactamase activities of SAWT, SACIP, and SAOXA were 78%, 57%, and 76%, respectively, for OE, EJ, and CL. These results are in a good agreement with previous report that medicinal plant extracts containing quercetin, naringenin, and catechin could act as β-lactamase inhibitors for enhancing antimicrobial activity (Boussoualim and Abderrahmane, 2011; Eumkeb et al., 2010). These β-lactamase inhibitors can directly interfere with β-lactamases or compete for the binding sites of β-lactamases (Catteau, et al., 2017).
In conclusion, the most significant finding was that medicinal plant extracts, including CJ, CL, EH, EJ, OE, and RM, potentiated the ampicillin activity against SAWT, SACIP, and SAOXA. The β-lactamase activities of SAWT, SACIP, and SAOXA were significantly reduced by 55-78%, 27-57%, and 65-76%, respectively, in the presence of CJ, CL, EH, EJ, OE, and RM, which were corresponded to the increased susceptibilities of SAWT, SACIP, and SAOXA treated with CJ, CL, EH, EJ, OE, and RM. Therefore, the medicinal plant extracts used in this study could be used as BLIs to enhance the ampicillin activity against SAWT, SACIP, and SAOXA. The antibiotic resistance mechanism-targeted approach can be a promising alternative for the control of MRSA. However, further study is needed to identify bioactive compounds in the medicinal plant extracts and elucidate their mechanisms for therapeutic use in combination with other antibiotics.
Abbreviations
Staphylococcus aureus ATCC 15564, SAWT; Clinically-isolated Staphylococcus aureus CCARM 3008, SACLI; Ciprofloxacin-induced Staphylococcus aureus ATCC 15564, SACIP; Oxacillin-induced Staphylococcus aureus ATCC 15564, SAOXA; Cleyera japonica, CJ; Carpinus laxiflora, CL; Euphorbia helioscopia, EH; Euscaphis japonica, EJ; Oenothera erythrosepala, OE; Rosa multiflora, RM.