Introduction
Materials and Methods
Chemicals
Preparation of P. communis Trinius root extract
Determination of total phenolic content and total flavonoid content
Determination of DPPH radical scavenging activity
Determination of hydrogen peroxide scavenging activity
Cell culture
Cell viability
Measurement of melanin production in B16F10 melanocytes
Measurement of tyrosinase inhibitory activity
Measurement of cellular elastase activity
UVB irradiation
Quantitative RT-PCR (qRT-PCR) analysis of MMP-1 and HAS-2 mRNA expression
Statistical analysis
Results
Total phenolic content and total flavonoid content
Radical scavenging activity of PCE
Inhibitory effect of PCE on tyrosinase activity in vitro
Effect of PCE on the viability of B16F10 melanocytes, HDFs, and HaCaT keratinocytes
Inhibitory effect of PCE on melanin production in B16F10 melanocytes
Inhibitory effect of PCE on cellular tyrosinase activity stimulated by α-MSH in B16F10 melanocytes
Suppressive effect of PCE on the mRNA expression of MMP-1 in HDFs
Suppressive effect of PCE on elastase activity in HDFs
Effect of PCE on the mRNA expression of HAS-2
Discussion
Introduction
The skin is a physical barrier to external stimuli, infection, and moisture loss (Lee et al., 2006). Keratinocytes form the major cell population in the epidermis and are involved in the development of the body’s barrier against environmental insults and water loss. Fibroblasts contribute to building the architecture of the extracellular matrix (ECM) in the dermis and produce various types of matrix metalloproteinases (MMPs), including MMP-1 and elastase (Freitas-Rodríguez et al., 2017). Skin aging is caused by chronological aging and/or photoaging, which is a premature type of skin aging caused by repeated and prolonged exposure to sunlight (Fisher et al., 2002). It has been postulated that reactive oxygen species (ROS) play a central role in both chronological aging and photoaging (Fisher et al., 2002). Excessive ROS production due to intrinsic or extrinsic factors, such as sunlight, increases MMP expression and transforming growth factor-β (TGF-β) signaling, which controls collagen homeostasis and affects wrinkle formation (Shin et al., 2019). ROS stimulate the α-melanin-stimulating hormone (α-MSH)-tyrosinase signaling pathway and consequently, increase melanin production from keratinocytes (Costin and Hearing, 2007). The cumulative structural and physiological alterations in the ECM caused by the aging process result in unattractive changes in skin appearance, such as folds and wrinkles (Ganceviciene et al., 2012). Wrinkles are a sign of loss of flexibility and dryness in the skin. Good skin health and beauty are considered to be representative of an overall high quality-of-life and a perception of good health in humans. In addition to invasive procedures, including chemical peelings and injection of botulinum toxin or filler, extensive efforts have been made to develop topical pharmacological agents (Shin et al., 2019). The development and standardization of plant-derived agents to inhibit skin aging is a key challenge for the cosmetics industry.
Phragmites communis (common reed) is a hydrophytic species inhabiting fresh swamps, riverbanks, and lakes; it grows well in temperate regions such as China, Korea, and Japan. Fresh and/or dried Phragmites rhizome has long been prescribed in Korea to relieve fever and vomiting and to facilitate diuresis (Park and Choi, 2016). A recent toxicological study showed that the aqueous extract of Phragmites rhizome had neither genotoxic nor mutagenic activities (Kim et al., 2019). Polysaccharides isolated from P. communis exerted anti-aging effects in a mouse model of aging (Miao et al., 2004). In addition, young leaves of P. communis played a role in the suppression of melanogenesis in B16F10 melanocytes (Sim et al., 2017). However, there are few reports on whether the ethanol extract of P. communis rhizomes (PCE) has anti-skin aging effects. In this study, we evaluated the total flavonoid and phenolic contents as well as the antioxidative potential of PCE based on ROS scavenging activities. The anti-melanogenic effects of PCE were evaluated through the inhibitory effects on tyrosinase in vitro and the suppression of melanin production in B16F10 melanocytes stimulated with α-MSH. In addition to the acceleration of melanogenesis, repeated ultraviolet (UV) irradiation damages the skin, resulting in a visible “solar scar” or wrinkles associated with photoaging (Helfrich et al., 2008). The anti-wrinkle potential of PCE was evaluated based on changes in the mRNA expression of MMP-1 and the elastase activity in human dermal fibroblasts (HDFs). In addition, to evaluate skin moisturization, changes in hyaluronic acid synthase-2 (HAS-2) gene expression in the presence or absence of UVB radiation in HaCaT keratinocytes were evaluated using qRT-PCR.
Materials and Methods
Chemicals
Ascorbic acid, arbutin, dimethylsulfoxide (DMSO), 2,2-diphenyl-1-picryl-hydrazyl radical (DPPH), mushroom tyrosinase, α-MSH, L-3,4-dihydroxyphenylalanine (L-DOPA), L-tyrosine, phenylmethylsulfonyl fluoride, phosphoramidon, retinol, N-succinyl-tri-alanyl-p-nitroanilide (STANA), TGF-β, and deionized distilled water were purchased from Sigma-Aldrich (St. Louis, MO, USA). Dulbecco’s modified Eagle’s medium (DMEM) was obtained from Welgene Inc. (Daegu, Korea). Fetal bovine serum was obtained from GenDEPOT (Katy, TX, USA). Absolute ethanol was obtained from DUKSAN (Seoul, Korea), penicillin/streptomycin was obtained from Gibco (Grand Island, NY, USA), and Trizol reagent was obtained from Invitrogen (Carlsbad, CA, USA). Unless otherwise indicated, all other chemicals were purchased from Sigma-Aldrich.
Preparation of P. communis Trinius root extract
The dried roots of P. communis Trinius were purchased from a local market in Suncheon-si (Korea) in January 2018. The material was identified by Dr. Y. K. Kim of the Korea Research Institute of Bio Science Inc. and stored in the dark at 4℃ until use. The dried roots (10 g) were mixed with 200 mL of 100% EtOH and extracted in a water bath at 60℃ for 4 h. The extract was filtered to remove any particulate matter, and evaporated under reduced pressure to obtain a powder, which was stored at -20℃ for further use in experiments. The yield of PCE was 4.2% (weight/weight).
Determination of total phenolic content and total flavonoid content
The total phenolic content was determined using the Folin–Ciocalteu method, as previously reported (So et al., 2019). Briefly, the extract was mixed with Folin–Ciocalteu’s phenol reagent (50 g/100 mL) and sodium carbonate (2 g/100 mL). After adjusting the final volume to 5 mL with deionized water, the reaction mixture was incubated at 25℃ for 30 min. The absorbance at 750 ㎚ was measured using a VICTOR X3 microplate reader (PerkinElmer, Waltham, MA, USA). The total phenolic content of PCE was calculated from a standard curve and expressed as ㎍ of gallic acid equivalent (GAE)/100 ㎎ of dried extract.
The total flavonoid content of PCE was determined using an aluminum chloride colorimetric method (So et al., 2019). Briefly, the extract was mixed with aluminum chloride hexahydrate (10 g/100 mL), 95% EtOH, and 1 M potassium acetate. After adjusting the final volume to 5 mL with deionized water, the reaction mixture solution was incubated at 25℃ for 40 min. The absorbance at 415 ㎚ was measured using a VICTOR X3 microplate reader. The total flavonoid content in the sample was calculated from the standard curve and expressed as ㎍ of quercetin equivalent (QE)/100 ㎎ of dried extract. All experiments were performed at least in triplicate.
Determination of DPPH radical scavenging activity
DPPH radical scavenging activity was determined as previously reported (So et al., 2019). Briefly, 100 µL reaction mixture containing different concentrations of PCE was reacted with 100 µL of 0.4 mM DPPH solution in 99% EtOH at 25℃ for 30 min. Decolorization of DPPH was measured at 540 ㎚ using a VICTOR X3 microplate reader. The half-maximal inhibitory concentration (IC50) for DPPH radical scavenging activity was calculated graphically by plotting the inhibition percentage against the tested PCE concentrations.
Determination of hydrogen peroxide scavenging activity
Hydrogen peroxide scavenging activity was determined as previously reported (So et al., 2019). Briefly, reaction mixtures containing different concentrations of PCE were reacted with an equal volume of 1.0 M H2O2 and 0.1 M phosphate buffer at 37℃ for 5 min. Then, 3-ethylbenzothiazoline-6-sulfonic acid and peroxidase were added. After the mixture was incubated at 37℃ for 10 min, changes in the absorbance at 405 ㎚ were measured using a VICTOR X3 microplate reader. The IC50 value for hydrogen peroxide scavenging activity was calculated graphically by plotting the percentage inhibition against the tested PCE concentrations.
Cell culture
Mouse melanoma B16F10 cells were obtained from the Korean Cell Line Bank (Seoul, Korea). Human dermal fibroblasts (HDFs) and human keratinocyte HaCaT cells were purchased from Gibco and CLS Cell Lines Service (Eppelheim, Baden-Württemberg, Germany), respectively. Cells were maintained in DMEM containing 10% fetal bovine serum and 1% penicillin/streptomycin in a humidified atmosphere of 5% CO2 at 37℃.
Cell viability
Cell viability was measured using the quantitative colorimetric 3-(4,5-dimethylthiazol-2-yl)-2,5-diphenyltetrazolium bromide (MTT) assay, as previously reported (Koo et al., 2017). B16F10 melanocytes in the exponential growth phase were seeded (1 × 104 cells/well) into 96-well tissue culture plates and treated with different concentrations (25-400 ㎍/mL) of PCE for 24 and 48 h. HDFs and HaCaT keratinocytes were seeded (1 × 104 cells/well) into 96-well tissue culture plates and treated with different concentrations (25-400 ㎍/mL) of PCE for 24 h. After incubation with MTT (150 ㎍/mL) for 4 h, the formed formazan crystals were dissolved in DMSO, and the optical density at 540 ㎚ was measured using a VICTOR X3 microplate reader. Cytotoxicity was expressed as a percentage of the untreated control cells.
Measurement of melanin production in B16F10 melanocytes
B16F10 melanocytes were seeded (2 × 105 cells/well) into 6-well cell culture plates and allowed to adhere overnight. The cells were then treated with α-MSH (100 nM) in the presence or absence of PCE (25-200 ㎍/mL) for 48 h. The cells were washed with ice-cold phosphate-buffered saline (PBS) and harvested using a cell scraper. After centrifugation (2500 × g, 25 ℃, 1 min), the pellets were dissolved in 1 N NaOH for 1 h at 60℃. The absorbance at 490 ㎚ was measured using a VICTOR X3 microplate reader.
Measurement of tyrosinase inhibitory activity
As previously reported (So et al., 2019), the inhibitory activity of PCE on tyrosinase was determined in a cell-free system using a colorimetric method with L-DOPA and L-tyrosine as the substrates. Different concentrations of PCE were added to the reaction buffer containing mushroom tyrosinase, and the enzymatic reaction was initiated after the addition of L-tyrosine or L-DOPA. After incubation at 37℃ for 15 min, the absorbance at 490 ㎚ was measured using a VICTOR X3 microplate reader. The IC50 value for tyrosinase activity was calculated from the graph by plotting the inhibition percentage against the tested PCE concentrations. To determine the inhibitory effect of PCE on cellular tyrosinase, B16F10 melanocytes were seeded (2 × 105 cells/well) into 6-well cell culture plates, and the cells were treated with α-MSH (100 nM) in the presence or absence of PCE (25-200 ㎍/mL) for 48 h. The cells were harvested, washed with PBS, and then lysed with lysis buffer containing 50 mM sodium phosphate buffer (pH 6.8), 1% Triton X-100, and 0.1 mM phenylmethylsulfonyl fluoride. After centrifugation (15,000 × g, 4℃, 30 min), tyrosinase activity of the cell supernatant (40 ㎍) was determined in a reaction mixture containing 40 µL of 100 mM sodium phosphate buffer (pH 6.8) and 160 µL of 10 mM L-DOPA for 1 h. Then, the absorbance at 490 ㎚ was measured using a VICTOR X3 microplate reader.
Measurement of cellular elastase activity
HDFs were seeded (2 × 106 cells/well) into 100-mm tissue culture dishes. Confluent cells were lysed with PRO-PREPTM protein extraction solution (Invitrogen, Seongnam, Korea), and the cell lysates were collected by centrifugation (15,000 × g, 4℃, 30 min). Equal volumes of cell lysates (each containing 100 ㎍ protein) were added to a 96-well plate. Then, PCE (25-200 ㎍/mL) and STANA (50 mM) were added, successively. After incubation at 37℃ for 90 min, the release of p-nitroaniline was measured at 405 ㎚ using a VICTOR X3 microplate reader. Elastase activity was expressed as a percentage of the untreated control.
UVB irradiation
UVC 500 UltraViolet Crosslinker (Amersham, Bucks, UK) was used for UVB irradiation, as previously described (Park et al., 2018). Briefly, HaCaT keratinocytes at approximately 90% confluence were switched to serum-free DMEM for 4 h. The cells were then covered with PBS and exposed to UVB light (34.06 s) for a total dose of 30 mJ/㎠. After washing with PBS, the cells were further cultured in DMEM containing 10% fetal bovine serum with or without PCE for 24 h. The control group treated in the same manner but did not receive UVB irradiation.
Quantitative RT-PCR (qRT-PCR) analysis of MMP-1 and HAS-2 mRNA expression
The mRNA expression of MMP-1 and HAS-2 was detected in HDFs and HaCaT keratinocytes, respectively. Total cellular RNA was extracted using TrizolTM reagent by the phenol-chloroform extraction method and reverse-transcribed to synthesize cDNA using a cDNA synthesis kit (ECDNA100, NanoHelix, Daejeon, Korea). Subsequently, PCR amplification was performed using a premier qPCR kit (PQL-S500, NanoHelix). The following reverse transcription conditions were used: 65℃ for 5 min, 42℃ for 60 min, and 95℃ for 5 min. The two-step PCR amplification conditions were 95℃ for 30 s, followed by 40 cycles of 95℃ for 5 s and 60℃ for 30 s to produce a melt curve. The primer sequences used were: 5'-AGT GGC CCA GTG GTT GAA AA-3' (forward) and 5'-CCA CAT CAG GCA CTC CAC AT-3' (reverse) for MMP-1, 5'-TGG ATC TCA TTC CTC AGC AG-3' (forward) and 5'-TCC ACA AAC TCA TGC AAC AA-3' (reverse) for HAS-2; and 5'-GTG GCA AAG TGG AGA TTG CC-3' (forward) and 5'-GAT GAT GAC CCG TTT GGC TCC-3' (reverse) for GAPDH.
Statistical analysis
Each experiment was repeated at least three times. The results were expressed as the mean ± standard error of the mean (SEM) and were analyzed using one-way ANOVA followed by Tukey’s test (Systat Software Inc., San Jose, CA, USA). A statistical probability value of p < 0.05 was considered significant.
Results
Total phenolic content and total flavonoid content
Phenolic acids and flavonoids are the major antioxidants found in plants (Cai et al., 2004). In addition to their broad biological functions, such as antioxidant and anti-inflammation activity, both types of compounds possess photoprotective activity because of their structural similarity to chemical filters (Saewan and Jimtaisong, 2015). The total phenolic content in PCE was determined to be 231.8 ± 0.001 ㎍ GAE/100 ㎎ of dried extract (n = 3) and that of flavonoid was 2.92 ± 0.007 ㎍ QE/100 ㎎ of dried extract (n = 3).
Radical scavenging activity of PCE
The antioxidant activities of up to 200 ㎍/mL PCE were evaluated in terms of the scavenging potential of DPPH radicals (Fig. 1A) and hydrogen peroxide (Fig. 1B). The IC50 values of PCE for the DPPH radical and hydrogen peroxide scavenging were 0.96 and 0.97 ㎎/mL, respectively.
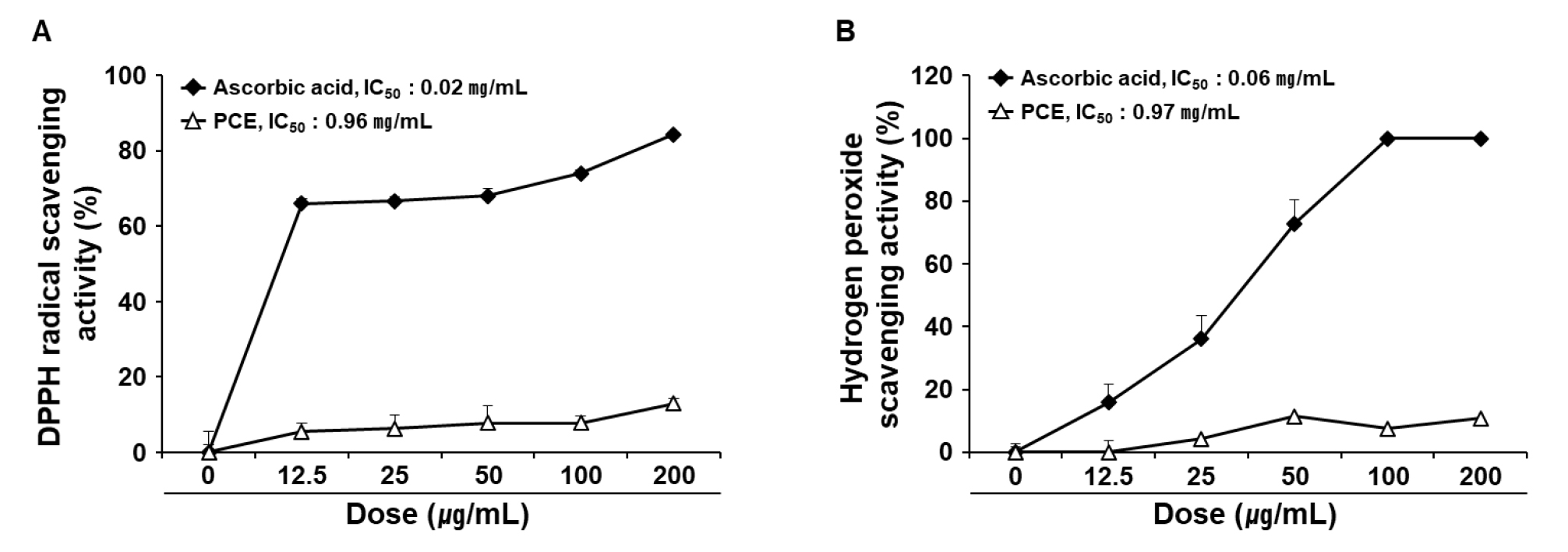
Fig. 1
Radical scavenging activity of PCE. The antioxidant activities of PCE were determined in terms of DPPH (A) and hydrogen peroxide (B) scavenging activities. Ascorbic acid was used as the control. IC50 values were determined using the regression equation. Data are presented as mean ± SEM (n = 4). DPPH, 2,2-diphenyl-1-picryl-hydrazyl-hydrate; IC50, half-maximal inhibitory concentration; PCE, ethanol extract of P. communis rhizome.
Inhibitory effect of PCE on tyrosinase activity in vitro
Suppression of tyrosinase activity is a key event in anti-melanogenesis. Polyphenols belong to the largest group of tyrosinase inhibitors and are potential skin-whitening agents (Chang, 2009). To determine the anti-melanogenic effect of PCE, we determined its inhibitory effect on tyrosinase in a cell-free system with different substrates, L-tyrosine and L-DOPA. The IC50 values of PCE on tyrosinase were 1.25 and 0.92 ㎎/mL for the substrates L-tyrosine (Fig. 2A) and L-DOPA (Fig. 2B), respectively.
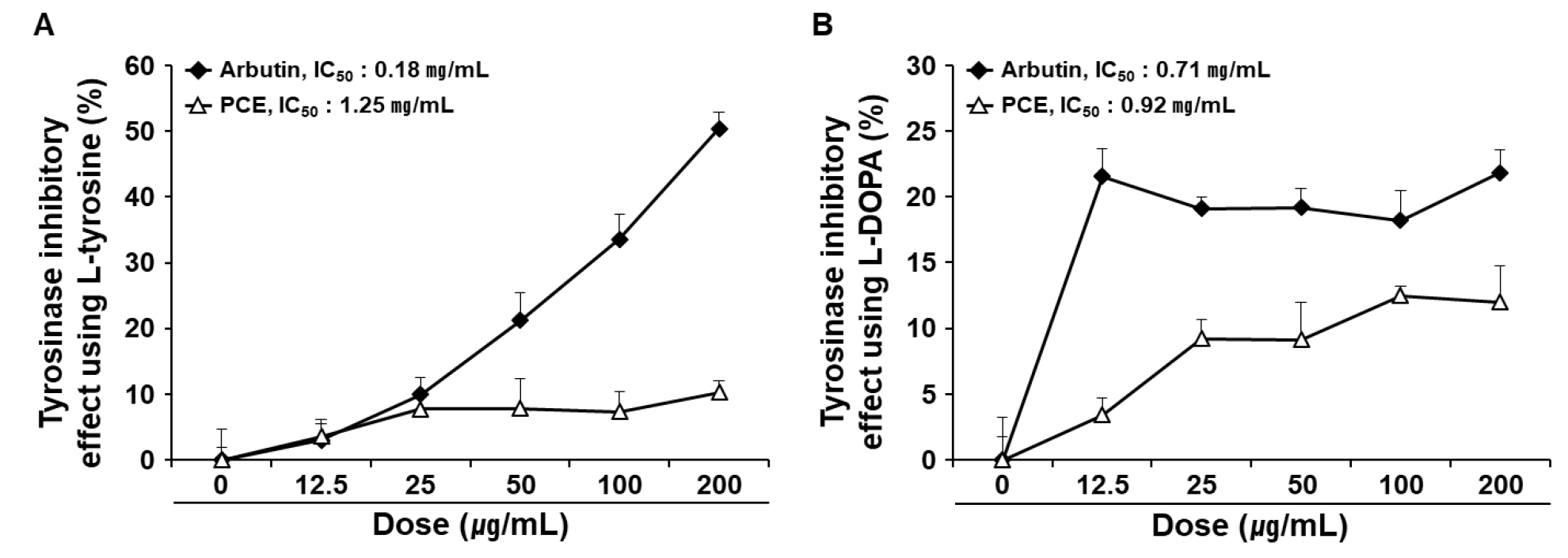
Fig. 2
Inhibitory effects of PCE on tyrosinase in a cell-free system. Tyrosinase regulatory activities were determined using a colorimetric method. L-tyrosine (A) and L-DOPA (B) were used as substrates for tyrosinase. IC50 values were determined using the regression equation. Data are means ± SEM (n = 4) and are expressed as a percentage of the untreated control. IC50, half-maximal inhibitory concentration; PCE, ethanol extract of P. communis rhizome.
Effect of PCE on the viability of B16F10 melanocytes, HDFs, and HaCaT keratinocytes
The MTT assay was performed to determine the cytotoxicity of PCE treatment in B16F10 melanocytes, HDFs, and HaCaT keratinocytes. Unlike the subsequent experiments performed in HDFs and HaCaT keratinocytes, the measurement of melanin production in B16F10 melanocytes requires treatment for 48 h of treatment. Thus, the effect of PCE on the viability of B16F10 melanocytes was also determined at 48 h. No significant cytotoxicity of PCE was observed up to 200 ㎍/mL in B16F10 melanocytes (Fig. 3A), HDFs (Fig. 3C), and HaCaT keratinocytes (Fig. 3D) at 24 h. However, at 400 ㎍/mL, PCE resulted in significant cytotoxicity in B16F10 melanocytes at 48 h (Fig. 3B). Thus, a PCE concentration below 200 ㎍/mL was used for the subsequent experiments.
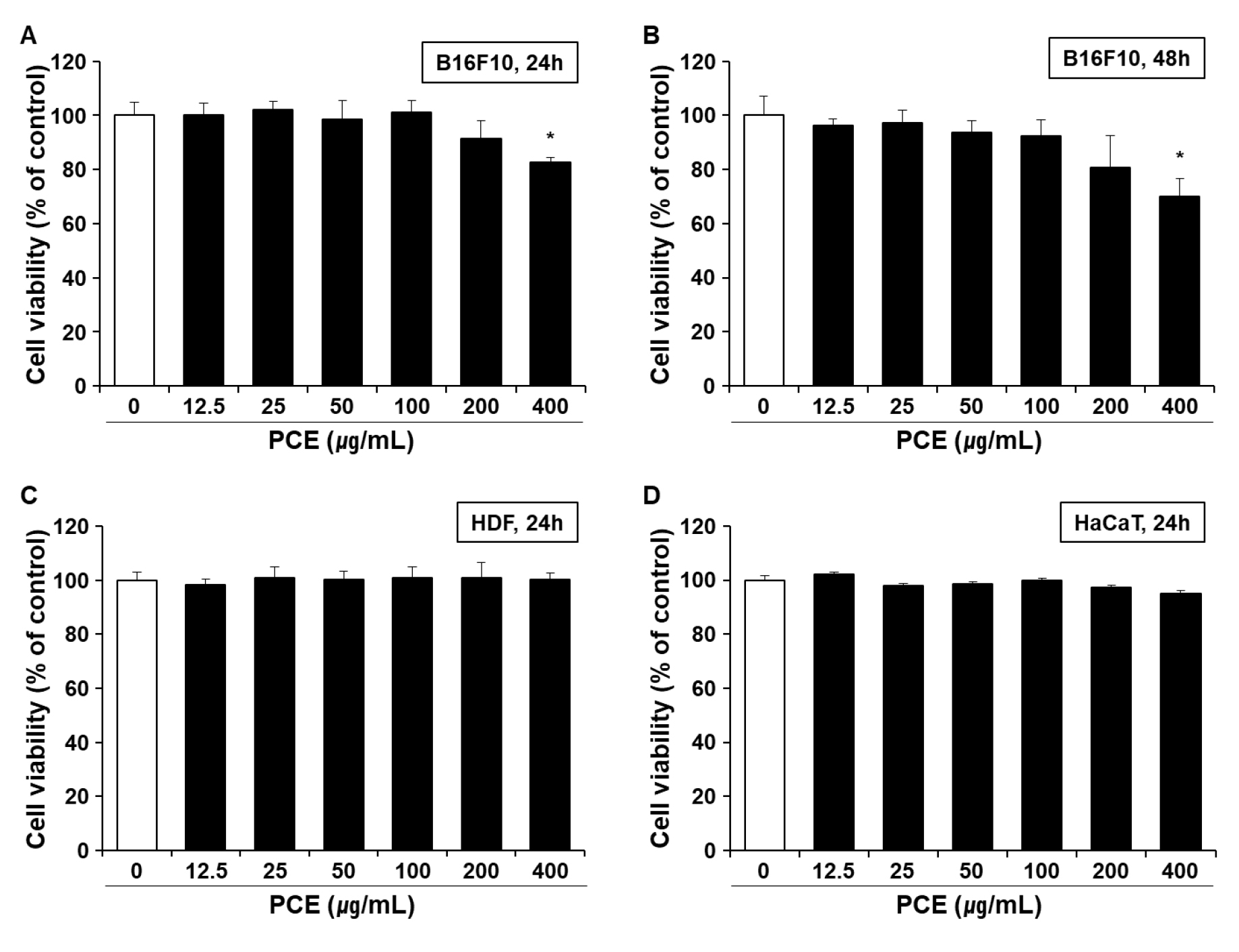
Fig. 3
Cytotoxicity of PCE. Melanocyte B16F10 cells were incubated with PCE for 24 h (A) and 48 h (B). Human dermal fibroblasts (HDFs) (C) and keratocytes (HaCaT) (D) were incubated in the presence of PCE for 24 h. The treatment dose of PCE ranged from 12.5 to 400 ㎍/mL. Cell cytotoxicity was determined using the MTT assay. Data are means ± SEM (n = 5) and are expressed as percentages of the untreated control. MTT, 3-(4,5-dimethylthiazol-2-yl)-2,5-diphenyltetrazolium bromide; PCE, ethanol extract of P. communis rhizome.
Inhibitory effect of PCE on melanin production in B16F10 melanocytes
α-MSH is a strong inducer of tyrosinase expression and is involved in the promotion of melanogenesis. The effect of PCE on melanin production in B16F10 melanocytes was determined in the presence of α-MSH (100 nM). Arbutin strongly inhibited tyrosinase and was thus used as a positive control. α-MSH stimulation increased melanin production in B16F10 melanocytes by 147 ± 1.49% compared with untreated control (p < 0.05). α-MSH-mediated melanin production was significantly suppressed in the presence 25 and 50 ㎍/mL PCE. However, the inhibitory effect of PCE on melanin production not observed at PCE concentrations above 100 ㎍/mL (Fig. 4A).
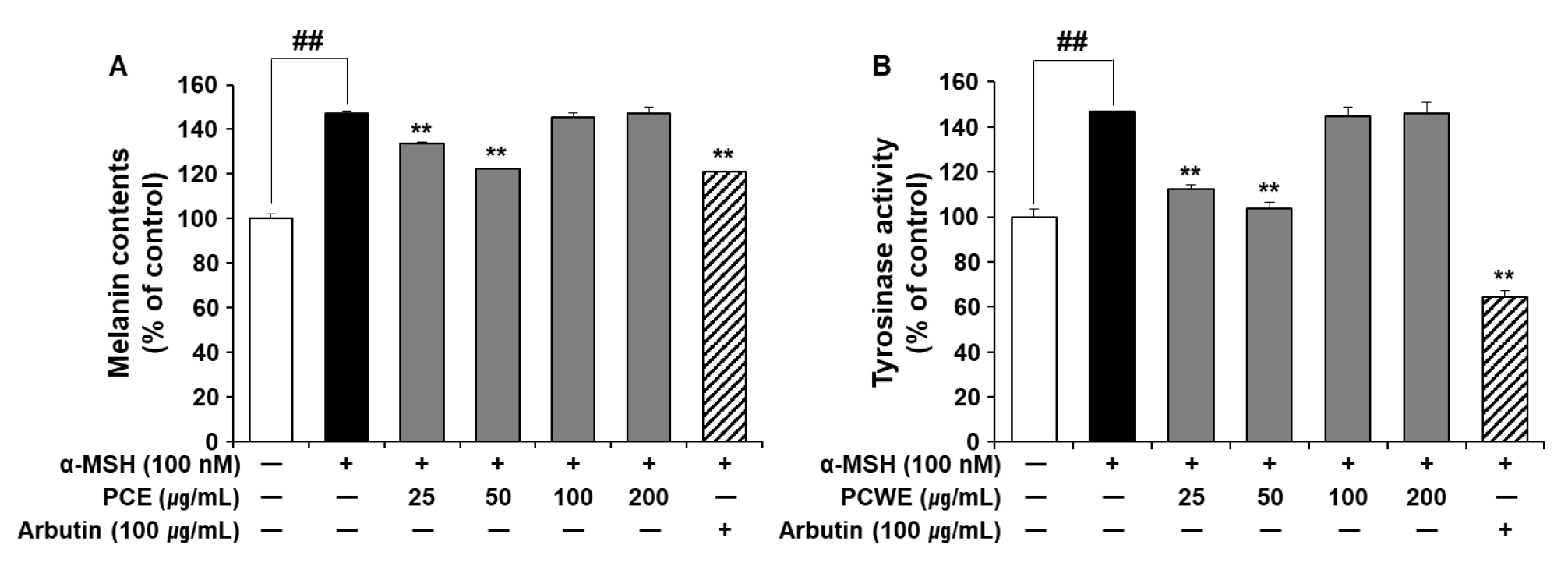
Fig. 4
Effects of PCE on melanin production and tyrosinase activity in B16F10 cells. B16F10 cells were exposed to α-MSH (100 nM) in the presence or absence of PCE (25–200 ㎍/mL) for 48 h. The content of melanin is expressed as a percentage of the untreated control cells (A). Cellular tyrosinase activity was determined by a colorimetric method and expressed as a percentage of the untreated control (B). Data are presented as mean ± SEM (n = 5). ##p < 0.01 vs. untreated control and **p < 0.01 vs. α-MSH-treated control. α-MSH, α-melanin-stimulating hormone; PCE, ethanol extract of P. communis rhizome.
Inhibitory effect of PCE on cellular tyrosinase activity stimulated by α-MSH in B16F10 melanocytes
PCE suppressed melanin production in α-MSH-stimulated B16F10 melanocytes (Fig. 4A). As shown in Fig. 3, PCE showed a direct inhibitory effect on tyrosinase activity. Therefore, we subsequently examined the effect of PCE on tyrosinase activity in α-MSH-stimulated B16F10 melanocytes (Fig. 4B). Consistent with the results of changes in the melanin content (Fig. 4A), significant tyrosinase inhibitory activity of PCE was observed at 25 and 50 ㎍/mL (p < 0.05).
Suppressive effect of PCE on the mRNA expression of MMP-1 in HDFs
Upon exposure to UVB, activator protein 1 (AP-1) is activated through various signaling pathways. The activation of AP-1 involves the upregulation of collagen-degrading enzymes (e.g., MMPs) and inhibition of collagen synthesis by counteracting the TGF-β signaling pathway (Fisher et al., 2002). MMP-1 is involved in the initiation of collagen cleavage (Fisher et al., 2009) and is thus a major contributor to degradation of the ECM. When the mRNA expression of MMP-1 in HDFs was determined via qRT-PCR, 25 and 50 ㎍/mL PCE was found to significantly downregulate MMP-1 expression (Fig. 5A, p < 0.05).
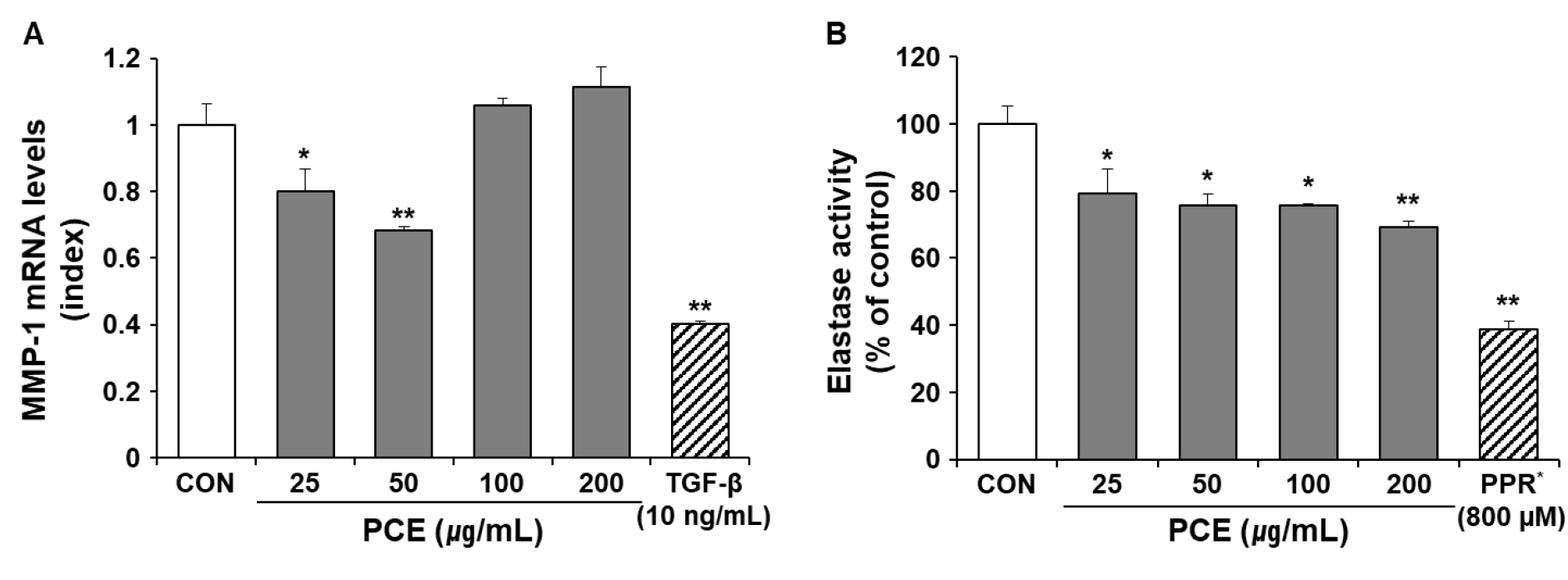
Fig. 5
Effects of PCE on mRNA expression levels of MMP-1 and elastase activity in HDFs cells. HDFs were treated with different doses of PCE for 24 h. The mRNA expression levels of the MMP-1 gene were determined using qRT-PCR and normalized to mRNA levels of GAPDH. Results are expressed as an index of the untreated control (A). Elastase activity was measured using STANA as a substrate and expressed as a percentage of the untreated control (B). TGF-β and PPR were used as positive controls for mRNA expression of MMP-1 and elastase activity, respectively. Data are presented as mean ± SEM (n = 5). ##p < 0.01 vs. CON and **p < 0.01 vs. α-MSH-treated control. α-MSH, α-melanin stimulating hormone; CON, untreated control; MMP-1, matrix metalloprotease-1; PCE, ethanol extract of P. communis rhizome; PPR, phosphoramidon; STANA, N-succinyl-tri-alanyl-p-nitroanilide; TGF-β, transforming growth factor-β.
Suppressive effect of PCE on elastase activity in HDFs
Skin fibroblasts secrete elastase, which belongs to the family of metalloproteinases. Skin fibroblast elastase plays a crucial role in the degradation of elastic fibers associated with wrinkle formation (Tsuji et al., 2001). Here, phosphoramidon, an inhibitor of skin fibroblast-derived elastase isolated from Pseudomonas aersa (Morihara and Tsuzuki, 1978), was used as a positive control. As shown in Fig. 5B, PCE significantly inhibited elastase activity in HDFs (p < 0.05).
Effect of PCE on the mRNA expression of HAS-2
Skin moisture is closely associated with water retention in the skin. Skin hyaluronan, or hyaluronic acid (HA), which is synthesized by the membrane-bound enzyme HAS, is a major contributor to water retention (Papakonstantinou et al., 2012). During skin aging, a significant reduction in the content of HA and the downregulation of HAS-2 have been identified (Papakonstantinou et al., 2012). To determine the moisturizing effect of PCE, we evaluated the mRNA expression of HAS-2 in HaCaT keratinocytes in the presence or absence of UVB. Retinol is a strong inducer of HAS gene expression in skin tissue (Li et al., 2017) and was used as the positive control. In basal conditions, the mRNA expression of HAS-2 did not increase at the applied PCE concentrations, except for 200 ㎍/mL (Fig. 6A). However, PCE treatment reversed the decrease in HAS-2 gene expression that followed UVB exposure (Fig. 6B). This result suggested that PCE did not directly upregulate the expression of HAS-2, but suppressed the UVB-mediated downregulation of HAS-2 gene expression.
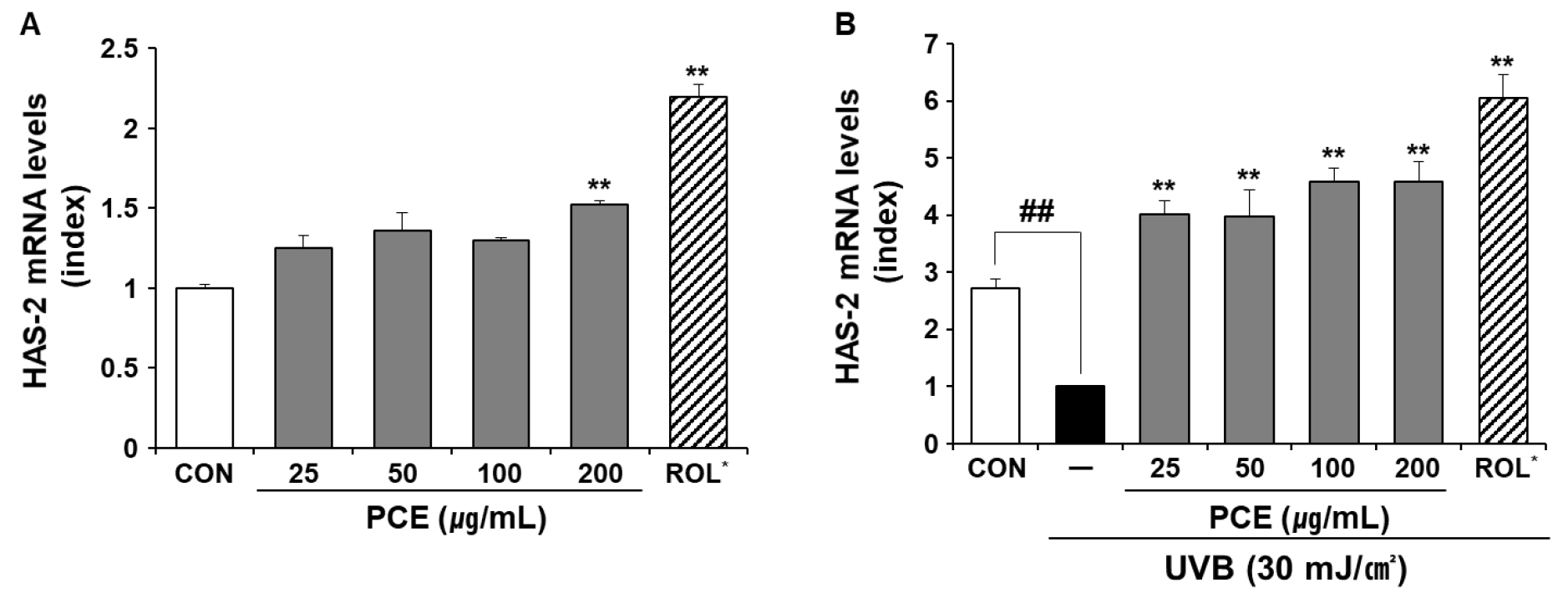
Fig. 6
Effect of PCE on the mRNA expression levels of HAS-2. UVB irradiation strength was set at 30 mJ/㎠. One group of HaCaT cells was treated with different concentrations of PCE (A), whereas the other group was treated with different concentrations of PCE followed by UVB irradiation (B). The mRNA expression levels of the HAS-2 gene were determined using qRT-PCR and normalized to mRNA levels of the GAPDH gene. ROL (10 ㎍/mL) was used as a stimulator of HAS-2 gene expression. Data are means ± SEM (n = 5) and expressed as an index of the respective control. ##p < 0.01 vs. CON and **p < 0.01 vs. UVB-treated control. CON, untreated control; HAS-2, hyaluronan synthase-2; PCE, ethanol extract of P. communis rhizome; ROL, retinol; UVB, ultraviolet B irradiation.
Discussion
The results of our study showed the value of PCE in the alleviation of skin aging. PCE possessed tyrosinase inhibitory activity and suppressed the α-MSH-mediated increase in melanin production in B16F10 melanocytes. PCE could not only suppress the mRNA expression of MMP-1, but also reduced the activity of elastase in HDFs. In addition, the UVB-mediated downregulation of HAS-2 gene expression in HaCaT keratinocytes was also effectively suppressed in the presence of PCE.
The conservation of smooth skin and youthful complexion is considered a very important part of an attractive appearance and beauty in Asia (Smit et al., 2009). Similar to other organs, the skin will age over time. Numerous approaches have been undertaken in the cosmetic industry to maintain healthy skin or prevent skin aging (Jadoon et al., 2015). Skin aging leads to the occurrence of folds and wrinkles due to atrophy of the dermis and deterioration of ECM organization (Helfrich et al., 2008). Prolonged and repeated exposure to sunlight, especially UVB, is a major cause of skin photoaging. Sunscreen provides a physical barrier against sunlight (e.g., UVB); however, simple protection against sunlight is not sufficient to maintain healthy skin. In some cases, synthetic skincare cosmetics may cause allergic reactions in the skin; however, natural skincare products may less frequently induce such reactions (Mukherjee et al., 2011). Alternatively, herbal extracts are good sources of bioactive compounds with anti-skin aging potential (Mukherjee et al., 2011). The suggested mechanisms of action of herbal extracts are associated with: 1) suppression of MMP activity; 2) antioxidant and free radical scavenging; or 3) increased hyaluronic acid content (Mukherjee et al., 2011).
In addition to the established use of Phragmitis rhizome as a traditional medicine (Park and Choi, 2016), we explored the anti-skin aging potential of PCE in this study. Antioxidants are recognized as important functional ingredients of cosmetic materials, with the ability to inhibit aging and skin pigmentation by ROS scavenging. PCE exhibits radical scavenging activities (Fig. 1) and contains phenolic acids and flavonoids. In addition to their various biological functions, phenolic acids present in PCE may function as a UVB blocker and attenuate skin damage caused by sunlight. Tyrosinase is the key enzyme in melanogenesis and is increased in the skin during UVB exposure (Gillbro and Olsson, 2011). Thus, tyrosinase inhibitors are the most characterized cellular targets of skin-whitening agents (Chang, 2009). At 25-200 ㎍/mL, PCE displayed tyrosinase inhibitory capacities in vitro and was more potent when L-DOPA was used as a substrate (Fig. 2). PCE possessed tyrosinase inhibitory activity and suppressed the α-MSH-mediated increase in melanin production in B16F10 melanocytes (Fig. 4). Collagen and elastin are the major constituents of the ECM that maintain skin architecture. The accelerated breakdown of the ECM impairs the dermal structure, resulting in the formation of wrinkles and the loss of skin elasticity. MMP-1 and elastase are involved in the degradation of ECM in the skin. The topical treatment of elastase inhibitors participates in the suppression of wrinkle formation caused by UVB exposure (Tsuji et al., 2001). The mRNA expression of MMP-1 and the activity of elastase were both reduced in the presence of PCE (Fig. 5); thus, these effects of PCE may contribute to the suppression of wrinkle formation. Finally, the maintenance of skin moisture, which is associated with hyaluronic acid content, is necessary to preserve the firmness and springiness of skin (Li et al., 2017). The levels of hyaluronic acid in the skin determine skin dryness and are frequently decreased by UVB exposure or normal aging. UVB exposure caused the downregulation of HAS-2 expression; however, this decrease in HAS-2 expression did not occur in the presence of PCE (Fig. 6). Therefore, PCE can contribute to the maintenance of hyaluronic acid in the skin. Interestingly, the suppressive effects of PCE on both cellular tyrosinase activity and the reduction of α-MSH-mediated melanin production were only observed with 25 and 50 ㎍/mL PCE (Fig. 4). The disappearance of the inhibitory activity of PCE above 50 ㎍/mL was also shown by the gene expression of MMP-1 in HDFs (Fig. 5A). However, the suppressive effect of PCE on the gene expression of HAS-2 was dose dependent. It should be noted that PCE shows differences in concentration or efficacy according to the type of skin cells used. The reason for this is unclear, and requires further examination.
In conclusion, PCE is a potential cosmetic ingredient owing to its suppressive effects on melanogenesis, wrinkle formation, and water loss in the skin. Further studies are warranted to identify the active compounds involved in the individual anti-aging pathways.