Introduction
Materials and Methods
ME preparation and preparation of extracts
Cell lines
Cell viability assay
NO assay
Total RNA extraction, cDNA synthesis, and reverse transcription polymerase chain reaction (RT-PCR) analysis
Immunoblotting analysis
Statistical analysis
Results
Identification of mushroom extracts (MEs)
Effects of MEs on LPS-induced cell proliferation in RAW264.7 cells
Effects of MEs on LPS-stimulated NO production in RAW264.7 cells
Effects of MEs on the LPS-induced increase in TNF-α, IL-6, and IL-1β mRNAs in RAW264.7 cells
Effects of MEs on LPS-induced iNOS, COX-2, and p65 expression in RAW264.7 cells
Discussion
Introduction
Mushrooms have a long history of use as a part of the human diet in many regions worldwide due to their organoleptic characteristics and nutritional value (Reid et al., 2017). They have a high protein content with almost all essential amino acids and are rich in diverse minerals and vitamin B, representing a good dietary source of these important nutrients (Robaszkiewicz et al., 2010). In addition to attracting a great deal of interest in many areas of foods, mushrooms are also useful for preventing diseases, such as hypertension, hypercholesterolemia, and cancer (Lull et al., 2005). Medicinal mushrooms have an established history of use in traditional Eastern therapies. Based on historical customs of North Eastern Asia, medicinal mushrooms such as Ganoderma lucidum (Reishi), Lentinus edodes (Shiitake), and Inonotus obliquus (Chaga) have been collected and hot-water-soluble fractions have been extracted (Wasser, 2002). However, appropriate preparation methods for mushrooms in food processing and pharmaceutical production to regulate cellular immune responses are still elusive. Furthermore, the types of mushrooms that have greater biological and pharmaceutical activities for enhancing the immune response have not yet been identified.
Inflammation is a biological defense response of the body to harmful stimuli, including tissue damage or pathogen infection (Park et al., 2018); this response is required for removal of damaging agents. Chronic inflammation can be differentially classified from acute inflammation and is generally associated with the development of diseases, such as atherosclerosis, asthma, rheumatoid arthritis, and cancer (Libby et al., 2002; Du et al., 2015).
During the inflammatory response, inflammatory cells such as macrophages secrete inflammatory mediators including nitric oxide (NO), tumor necrosis factor-α (TNF-α), interleukin (IL)-6, and IL-1β (Mann et al., 2005). Lipopolysaccharide (LPS), a well-known endotoxin, activates the transcription factor nuclear factor-κB (NF-κB) and induces the expression of inflammatory cytokines, such as inducible NO synthase (iNOS) and cyclooxygenase-2 (COX-2), resulting in the production of inflammatory mediators (Guzik et al., 2003; Yi et al., 2017). Currently, many anti-inflammatory drugs cause side effects such as gastritis, nephritis, and cardiac disorders. Accordingly, many studies have attempted to identify safer anti-inflammatory substances among commonly consumed dietary foods.
In this study, we aimed to investigate whether mushroom extracts possessed anti-inflammatory effects. Primarily, we considered that mushroom extracts should be easily prepared and ready to eat. For this purpose, the solvents were applied either water or ethanol. To this end, we examined the production of LPS-induced NO using RAW264.7 cells and evaluated the mRNA levels of cytokines and protein accumulation of iNOS and COX-2 following modification of NF-κB protein levels. Our data provide insights into the utility of mushroom extracts (MEs) in the regulation of immune responses and anti-inflammatory activities.
Materials and Methods
ME preparation and preparation of extracts
Mushrooms were purchased from Yeoncheon, South Korea in June, 2014. The dried fruiting body at 50℃ for 3 days of 22 species of mushroom was placed into a flask and extracted three times with 10 volume of 70% ethanol or water at room temperature for 1 day, and then filtered through a PTEE syringe. The filtrate was condensed by evaporation under reduced pressure at 50℃ and freeze-dried, and the weight was measured.
Cell lines
RAW264.7 macrophages were obtained from the Korean Cell Line Bank (KCLB NO. 40071, Seoul, Korea). The cells were grown in Dulbecco’s modified Eagle’s medium (Gibco Life Technologies, Carlsbad, CA, USA) containing 10% fetal bovine serum, 50 ㎍/㎖ penicillin, and 50 ㎍/㎖ streptomycin in a 5% CO chamber at 37℃.
Cell viability assay
RAW264.7 cells were seeded in 96-well plates at a density of 1 × 104 cells/well. The cultured cells were treated with 1 ㎍/㎖ LPS for 4 h and MEs were then added in dimethylsulfoxide (DMSO) at various concentrations. Solvent control cells were treated with LPS alone. After 24 h of culture with or without samples, MTS working solution (Promega, CA, USA) was added into the culture plates for 4 h at 37℃. The absorbance of individual wells was measured at 540 nm using a microplate reader (Molecular Devices, CA, USA), and cell viability was calculated as the percent absorbance relative to LPS-treated control cells.
NO assay
The level of NO production in RAW264.7 cell supernatants was determined using Griess reagent (Promega), according to the manufacturer’s instructions. Briefly, the extracts were treated as described for the cell viability assays, and the cell culture supernatants were then collected in 96-well plates for nitrite assays. The absorbance was measured at 540 nm using a microplate reader (Molecular Devices, CA, USA). The concentration of nitrite was calculated from a standard curve.
Total RNA extraction, cDNA synthesis, and reverse transcription polymerase chain reaction (RT-PCR) analysis
Total RNA was isolated from RAW264.7 cells using TRIzol Reagent (Invitrogen, NY, USA), and 1 ㎍ of RNA was used for cDNA synthesis. RT-PCR was performed where each cDNA was amplified using gene-specific primers (Table 1) and a Maxime PCR RedMix kit (iNtRON Biotechnology, Seongnam, Korea). GADPH expression was used to normalize the expression value in each sample and relative expression values were determined against the average value of LPS-treated samples without MEs using ImageJ software.
Table 1. The pro-inflammatory gene primer used for RT-PCR
Immunoblotting analysis
RAW264.7 cells were collected and lysed in ice-cold RIPA buffer (20 mM Tris-HCl, pH 7.5, 150 mM NaCl, 1 mM Na2-ethylenediamintetraacetic acid, 1 mM ethylene glycol-bis[β-aminoethyl ether]-N,N,N',N'-tetraacetic acid, 1% NP-40, 1% sodium deoxycholate, 2.5 mM sodium pyrophosphate, 1 mM β-glycerophosphate, 1 mM Na3VO4, and 1 ㎍/㎖ leupeptin) for 30 min, and protein levels were measured using the BCA assay (Thermo Scientific, Rockford, IL, USA). The extracted protein was separated using 12% sodium dodecyl sulfate-polyacrylamide gel electrophoresis and then transferred onto nitrocellulose membranes (GE Healthcare, Chalfont St. Giles, UK). After blocking with Tris-buffered saline containing 5% nonfat dry milk and 0.1% (w/v) Tween 20, the membranes were immunoblotted with specific antibodies and then subjected to enhanced chemiluminescence (ECL kit; GE Healthcare). The relative band intensities were determined using chemiluminescence (Davinch-chemi Chemiluminescence Imaging System; CAS-400SM; Seoul, Korea). The relative protein intensity of each sample (200 ㎍/㎖) was calculated by normalizing against the intensity of β-actin as a loading control.
Statistical analysis
Unless otherwise indicated, values represent the mean ± standard deviation (SD) of data obtained from triplicate experiments. Statistical analysis was performed using Student’s t-tests.
Results
Identification of mushroom extracts (MEs)
To obtain MEs, either water or 70% ethanol, herein referred to simply as ethanol, was used as the extraction solvent based on the consideration that MEs should be directly edible. We chose the dried fruiting body of the mushrooms, and extraction was performed at room temperature for 1 day. The MEs are listed in Table 2.
Table 2. Relative effects of mushroom extracts on LPS-treated RAW264.7 cells
Effects of MEs on LPS-induced cell proliferation in RAW264.7 cells
To examine the effects of cell viability in the presence of MEs, we performed MTS assays using RAW264.7 cells, which were derived from mouse leukemic monocyte macrophages. The MTS assay is commonly used for analyze cell cytotoxicity and its result is correlated with cell proliferation. Cell proliferation was not changed by LPS (Table 3) or ME treatment in the absence of LPS (data not shown). LPS-induced cell proliferation was not altered by around 36 different spices of MEs (Table 2, Table 3). However, some MEs caused reduced cell proliferation under the LPS treatment. For example, Isaria japonica Yasuda (I. japonica) and Inonotus obliquus (Fr.) Pilát (I. obliquus) ethanol extracts showed only 53.64% ± 9.10% and 58.66% ± 2.76% cell proliferation, respectively, relative to the control, indicating that these MEs may exhibit cytotoxicity in RAW264.7 cells (Table 3). Indeed, even when using a relatively low concentration of 100 ㎍/㎖ I japonica and I. obliquus MEs, cell growth reached only 54.81% ± 6.77% and 58.77% ± 5.35%, respectively (Table 3). Thus, although some MEs affected cell proliferation, most MEs yielded 100% or higher viability, indicating that these MEs did not exert cytotoxic effects in RAW264.7 cells.
Table 3. Table 3. The mushroom extracts effect of cell proliferation and NO release content
Effects of MEs on LPS-stimulated NO production in RAW264.7 cells
To observe LPS-induced NO production in RAW264.7 cells in the presence of MEs, cells were treated with LPS (1 ㎍/㎖) for 4 h and then treated with DMSO or various MEs for 24 h. After 24 h of incubation, the NO levels in the cellular supernatants were assessed by Griess assays. Treatment with LPS increased NO production (30.68 ± 2.17 μM) compared with that in the absence of LPS (2.17 ± 0.02 μM; Table 2, Table 3). In contrast, post-treatment with 200 ㎍/㎖ MEs resulted in reduced LPS-induced NO generation (Table 3). Specifically, MEs prepared using Pleurotus cornucopiae (paulet) Rolland var. citrinopileatus (Sing.) Ohira, and I japonica extracts with water as well as those prepared using I. obliquus t and U. esculenta extracts with ethanol reduced NO contents to 3.75 ± 0.69 μM, 4.25 ± 0.74 μM, and 2.17 ± 0.35 μM, 2.00 ± 1.10 μM, respectively, similar to that without LPS (2.17 ± 0.02 μM; Table 3). We further tested NO production in the presence of 100 ㎍/㎖ MEs to investigate the effects of a lower concentration of MEs. Notably, 100 ㎍/㎖ MEs yielded results similar to those above, except that the NO concentration was higher than that induced by 200 ㎍/㎖ MEs (Table 3), indicating that LPS-induced NO contents were reduced in an ME concentration-dependent manner. Statistically significant differences were calculated by Student’s t-test, p < 0.05 (*), p < 0.01(**), and p < 0.001 (***). As NO is a signaling molecule involved in acute and chronic inflammatory diseases, NO levels are used to be representing inflammation. These results suggest that extracts from several mushrooms including I japonica, I. obliquus, U. esculenta, and P. linteus exerted anti-inflammatory effects via reduction of NO in LPS-stimulated RAW264.7 cells.
Before further investigating the anti-inflammatory effects of MEs, we summarized the above results for cell proliferation and NO reduction. The values were obtained by comparing the changes in cell proliferation and NO contents between 200 ㎍/㎖ MEs and 100 ㎍/㎖ MEs (Table 2). Thus, we chose two ME candidates: I. japonica water extract and U. esculenta ethanol extract due to that the change rate of cell growth was close to 1 for no change (i.e less cytotoxic), and the change rate of NO content was around 0.2 for 80% reduction on the treatment of 200 ㎍/㎖ MEs (i.e effective for NO reduction) (Table 2).
Effects of MEs on the LPS-induced increase in TNF-α, IL-6, and IL-1β mRNAs in RAW264.7 cells
To evaluate the anti-inflammatory effects of these two MEs, we analyzed the expression of inflammatory marker genes by reverse transcription PCR. Because murine macrophages produce pro-inflammatory cytokines, such as TNF-α, IL-6, and IL-1β, during the inflammatory response, LPS treatment increases the these transcripts (Mann et al., 2005). Given the screening results for the chosen two MEs, RAW264.7 cells were pre-incubated for 4 h with 1 ㎍/㎖ LPS and cultured 24 h more with the indicated concentrations (50–200 ㎍/㎖) of MEs. LPS treatment induced TNF-α, IL-6, and IL-1β gene expression. However, LPS-induced accumulation of TNF-α, IL-6, and IL-1β mRNA was significantly reduced after treatment with both I. japonica extracts (Fig. 1) and U. esculenta extracts (Fig. 2). In addition, reduction of the these transcripts was much more apparent following treatment with the high concentration (200 ㎍/㎖) of MEs compared with that after treatment with a low concentration (50 ㎍/㎖) of MEs (Fig. 3B–D and Fig. 4B–D). Statistically, the values revealed that the difference were significant with p < 0.05 (*), p < 0.01(**), and p < 0.001(***) by Student’s t-test. This result indicated that both I. japonica and U. esculenta extracts suppressed LPS-mediated transcription of pro-inflammatory cytokines.
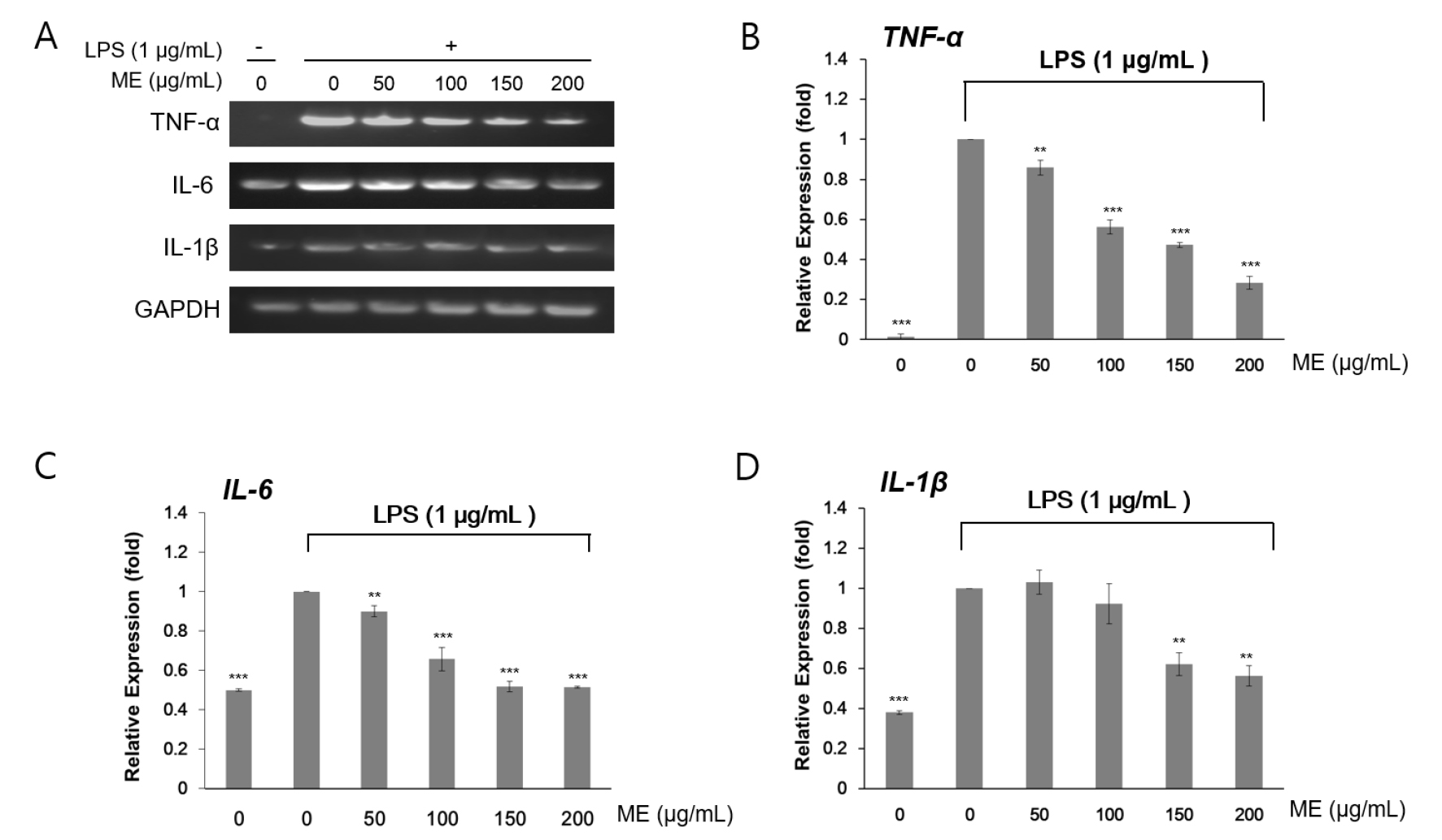
Fig. 1.
Effects of water extracts of I. japonica on pro-inflammatory gene expression in RAW264.7 cells. (A) The expression levels of TNF-α, IL-6, and IL-1β mRNAs were determined by RT-PCR analysis. Cells were treated with 1 μg/mL LPS and then I. japonica extracts at various concentrations for 24 h. GADPH was used as an internal control. The expression levels of TNF-α (B), IL-6 (C), and IL-1β (D) were determined by densitometry analysis. Values are expressed as the mean ± SD of four determinations. Significance was determined using Student’s t-tests: *p < 0.05, **p < 0.01, and ***p < 0.001 versus cells treated with LPS alone.
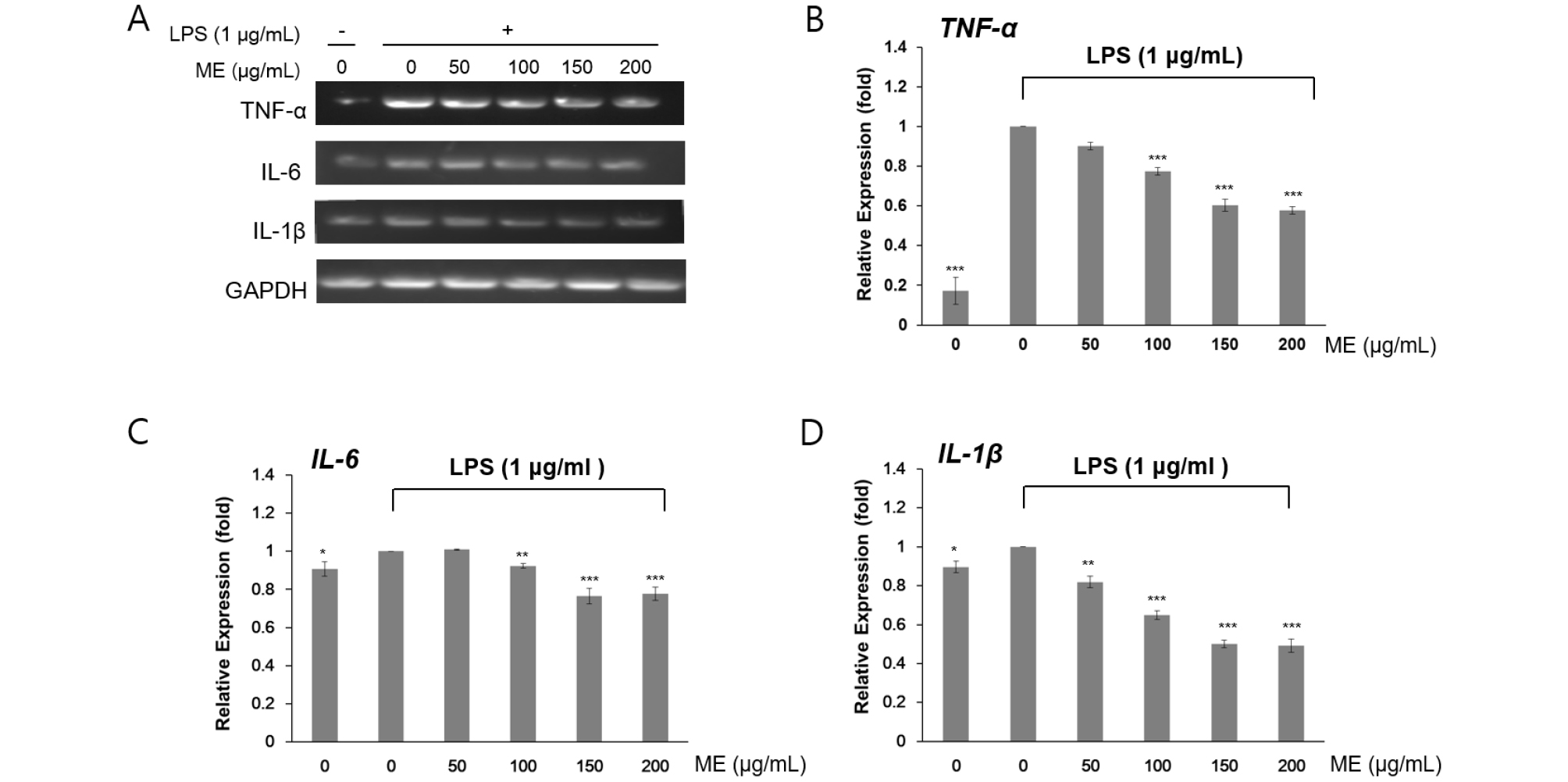
Fig. 2.
Effects of ethanol extract of U. esculenta on pro-inflammatory gene expression in RAW264.7 cells. (A) The expression levels of TNF-α, IL-6, and IL-1β mRNA was determined by RT-PCR analysis. Cells were treated with 1 ㎍/㎖ LPS and then U. esculenta extracts at various concentrations for 24 h. GADPH was used as an internal control. The expression levels of TNF-α (B), IL-6 (C), and IL-1β (D) were determined by densitometry analysis. Values are represented as the mean ± SD of four determinations. Significance was determined using Student’s t-tests: *p < 0.05, **p < 0.01, and ***p < 0.001 versus cells treated with LPS alone.
Effects of MEs on LPS-induced iNOS, COX-2, and p65 expression in RAW264.7 cells
Next, we examined whether I. japonica and U. esculenta extracts suppressed the expression of transcription factors related to the inflammatory process, e.g., iNOS and COX-2. iNOS and COX-2 are key pro-inflammatory proteins, and the expression of these proteins is controlled by signaling downstream of NF-κB (T and A, 2006). To test this hypothesis, we investigated iNOS and COX-2 protein expression and p65 protein modification by western blotting following treatment with MEs. p65, a subunit of NF-κB, undergoes phosphorylation (p-P65) to activate gene expression, including COX-2 and iNOS genes (T and A, 2006).
LPS treatment increased iNOS and COX-2 expression by inducing the inflammatory response in RAW264.7 cells. As expected, immunoblotting analyses indicated that iNOS and COX-2 expression and post-translation modification of p65 by LPS pretreatment were inhibited after incubation with I. japonica (Fig. 3) and U. esculenta (Fig. 4) MEs. In addition, p65 protein abundance was slightly increased by LPS, whereas p65 protein phosphorylation was dramatically decreased (Fig. 3D and Fig. 4D). These results demonstrated that both I. japonica and U. esculenta extracts suppress LPS-stimulated p65 phosphorylation and affected COX-2 and iNOS protein abundance. Because the pro-inflammatory enzyme iNOS is responsible for NO production, inhibition of iNOS protein accumulation resulted in reduced NO content following treatment with I. japonica and U. esculenta extracts.
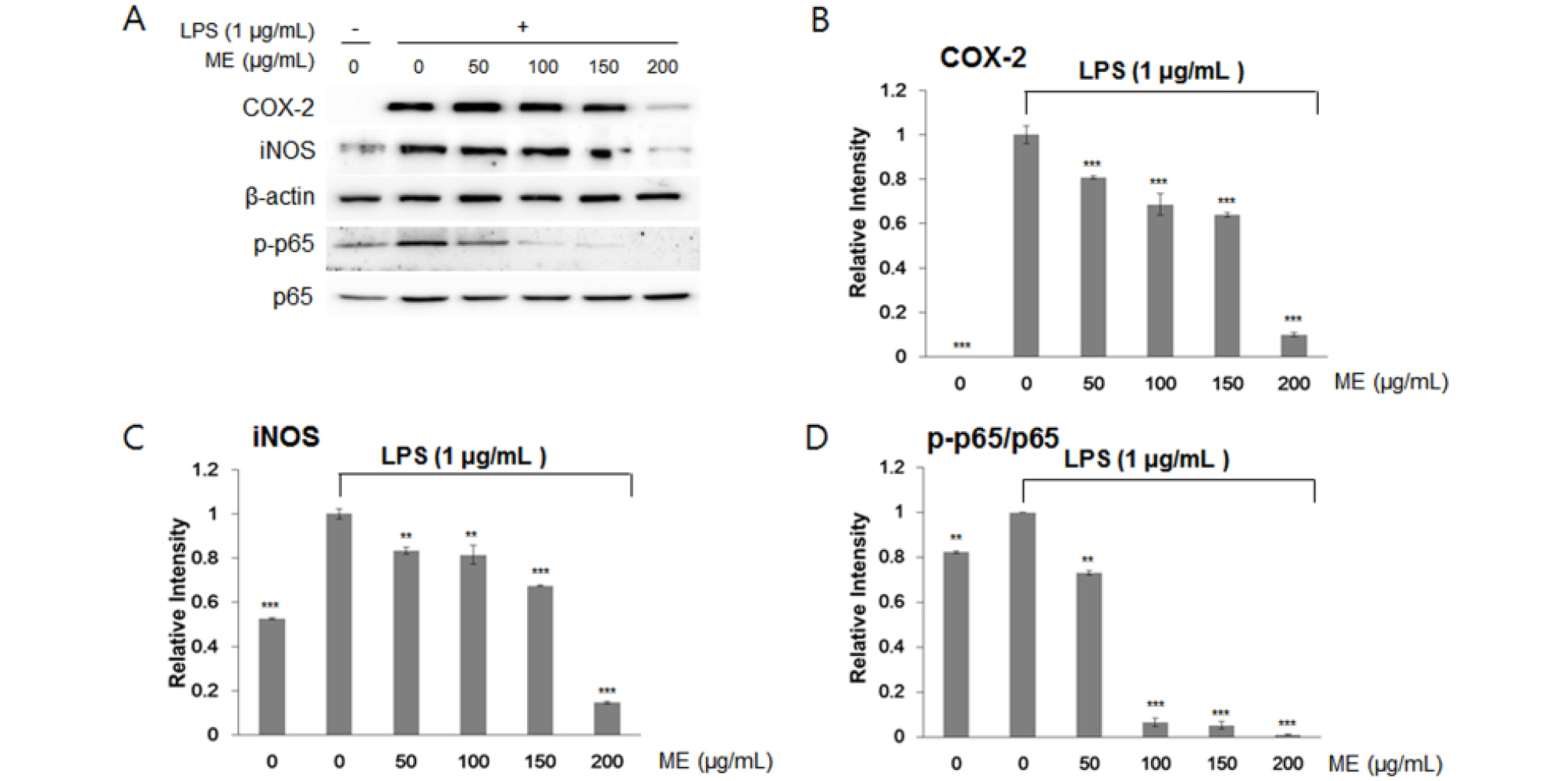
Fig. 3.
Effects of water extracts of I. japonica on the NF-κB pathway in RAW264.7 cells. (A) COX-2, iNOS, and p65 protein abundance was determined by a western blotting. Relative protein abundance of IL-6 (B) and IL-1β (C) and the level of Phosphorylated p65 (D), and were determined by densitometry analysis. Values are expressed the mean ± SD of four determinations. Significance was determined using Student’s t-tests: *p < 0.05, **p < 0.01, and ***p < 0.001 versus cells treated with LPS alone.
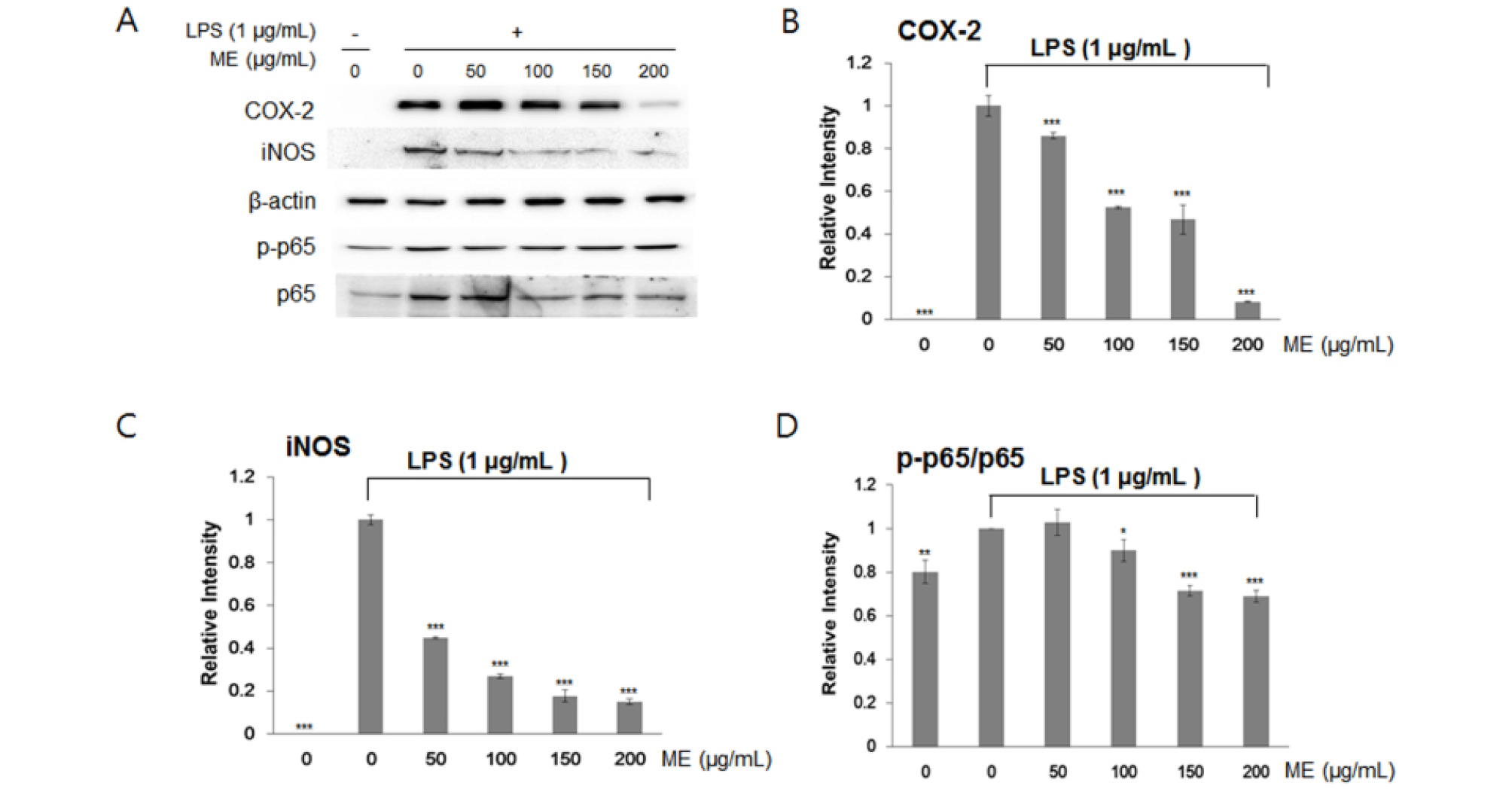
Fig. 4.
Effects of ethanol extracts of U. esculenta on the NF-κB pathway in RAW264.7 cells. (A) COX-2, iNOS, and p65 protein levels were determined by western blot analysis. IL-6 (B) and IL-1β (C) protein levels were determined by densitometry analysis. Phosphorylated p65 levels were determined (D). Values are expressed as the mean ± SD of four determinations. Significance was determined using one-factor t-tests: *p < 0.05, **p < 0.01, and ***p < 0.001 versus cells treated with LPS alone.
Discussion
Macrophages play important roles in inflammation through the production of several pro-inflammatory molecules, including NO. Uncontrolled, excessive NO has been shown to be associated with a range of inflammatory diseases, including arteriosclerosis, ischemic reperfusion, hypertension, and septic shock (Alvarez and Evelson, 2007; Pacher et al., 2007). Recent studies have demonstrated that plant foods, including fruits, vegetables, and medicinal herbs, are excellent sources of antioxidant molecules that effectively inhibit the inflammatory process by influencing different molecular targets (Tsai et al., 2007).
Edible mushrooms are used as functional foods due to their high content of bioactive compounds. However, their anti-inflammatory properties and the effects of processing steps on their bioactivity have not been systemically investigated. The anti-inflammatory effect of mushrooms of water extracts were reported using Sparassis cispa (Wulfen) Fr. (Kim et al., 2012), Ganoderma lucidum (Curtis) P. Karst (Kuo et al., 2006) and Grifola frondosa (Dicks.) Gray (Radic et al., 2010). The anti-inflammatory effect of mushrooms of ethanol extracts were reported Pleurotus eryngii (DC.) Quél (Lin et al., 2014), Agaricus bisporus (J.E. Lange) Imbach (Gunawardena et al., 2014; Taofiq et al., 2015), Pleurotus ostreatus (Jacq.: Fr.) P. Kumm (Taofiq et al., 2015), Hypsizygus marmoreus (Peck) H.E. Bigelow (Chien et al., 2016), Inonotus obliquus (Fr.) Pilat (Park et al., 2005; Van et al., 2009; Ma et al., 2013), Lentinula edodes (Berk.) Pegler (Gunawardena et al., 2014), Ganoderma lucidum (Curtis) P. Karst (Yoon et al., 2013). Accordingly, in this study, we found that MEs effectively suppressed LPS-induced inflammation. In addition, we showed that water extracts of I. japonica and ethanol extracts of U. esculenta inhibited the LPS-induced increase in NO production and pro-inflammatory cytokine expression in RAW264.7 cells.
LPS, a component of the outer membrane of gram-negative bacteria, activates macrophages and promotes the synthesis and release of molecules involved in the inflammatory response, such as cytokines, NO, and pro-inflammatory enzymes (O'Connellet al., 1998). We observed that MEs prepared using water or ethanol effectively reduced LPS- induced NO accumulation in RAW264.7 macrophages, suggesting that the MEs mitigated the pro-inflammatory reaction. Accumulating evidence has shown that NO is directly involved in the development of inflammation (Aktan, 2004; Pacher et al., 2007). In particular, high concentrations of NO, synthesized by iNOS, can mediate inflammation and cause cell death by triggering apoptosis (Wink and Mitchell, 1998).
Interestingly, both water extracts of I. japonica and ethanol extracts of U.esculenta elicited changes in TNF-α, IL-1β, and IL-6 mRNA expression and in accumulation of iNOS and COX-2 enzymes, and they modulated p65 phosphorylation, suggesting that these MEs could inhibit the expression of the pro-inflammatory cytokines and modulate NF-κB signaling to control NO. Therefore, the findings of our study reveal that water extracts of I. japonica and ethanol extracts of U. esculenta possess a protective effect in LPS-induced RAW264.7 cells. In addition, these results support that mushrooms are healthy foods and sources of medicinal components. One benefit of these MEs is that they can be prepared easily, and this benefit might contribute to developing healthy beverages or drugs to prevent or treat inflammatory diseases.